– AN ONGOING PROJECT.
Introduction.
Since the discovery of the first incontrovertible volcanic component of the Cape Granite Suite (CGS), the Postberg ignimbrites (Scheepers and Nortjè 2000) (Fig. 1a) and the description of the previously named “Saldanha quartz porphyry”, north of Saldanha Bay as part of the volcanics (Scheepers and Schoch 2006), much emphasis has been placed on the investigation of these rocks (Clemens and Stevens 2016, 2017 for example). Volcanic and subvolcanic features related to the CGS however, have been recognized over a long time period and in a variety of showings.
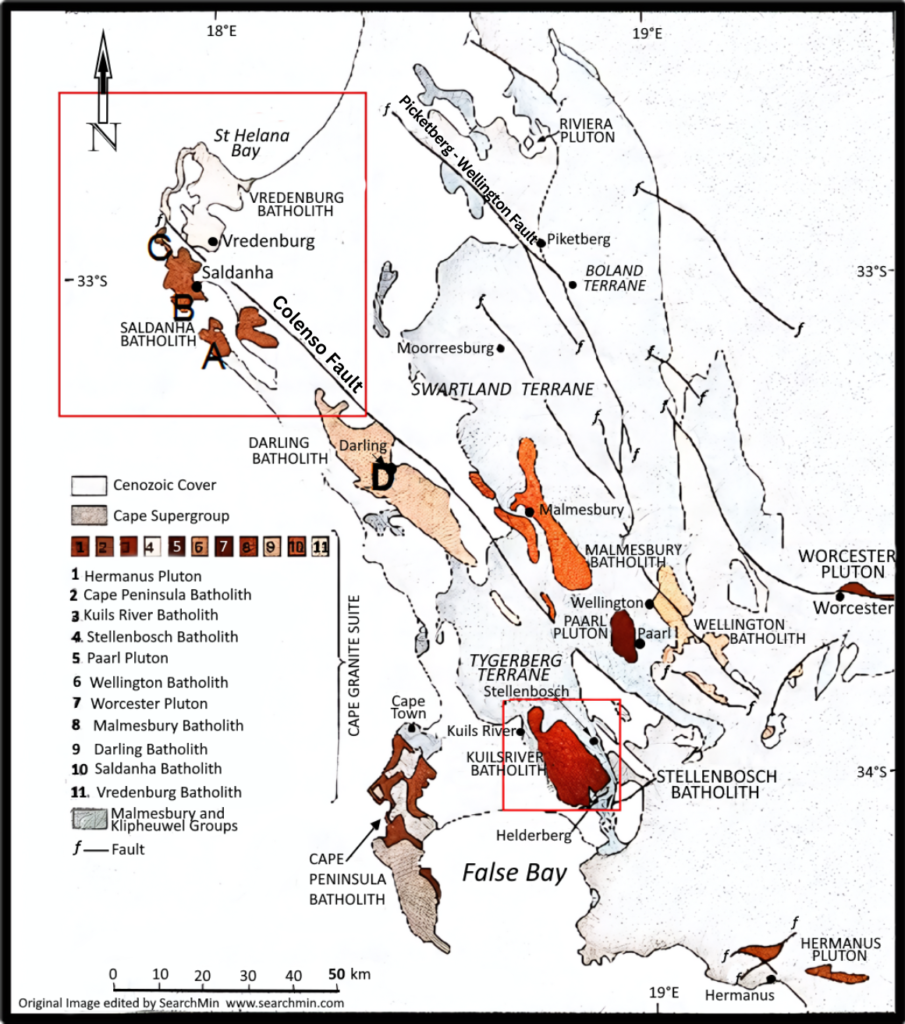
A: Postberg Ignimbrite.
B: Volcanic and Hypabyssal rocks north of Saldanha Bay.
C: Cataclasites of Northwest Bay (Schoch 1962) and
D: breccia pipes near Darling (Scheepers and Cuney 1990). Red Boxes outlines areas of ongoing (current) work on volcanic and hypabyssal rocks.
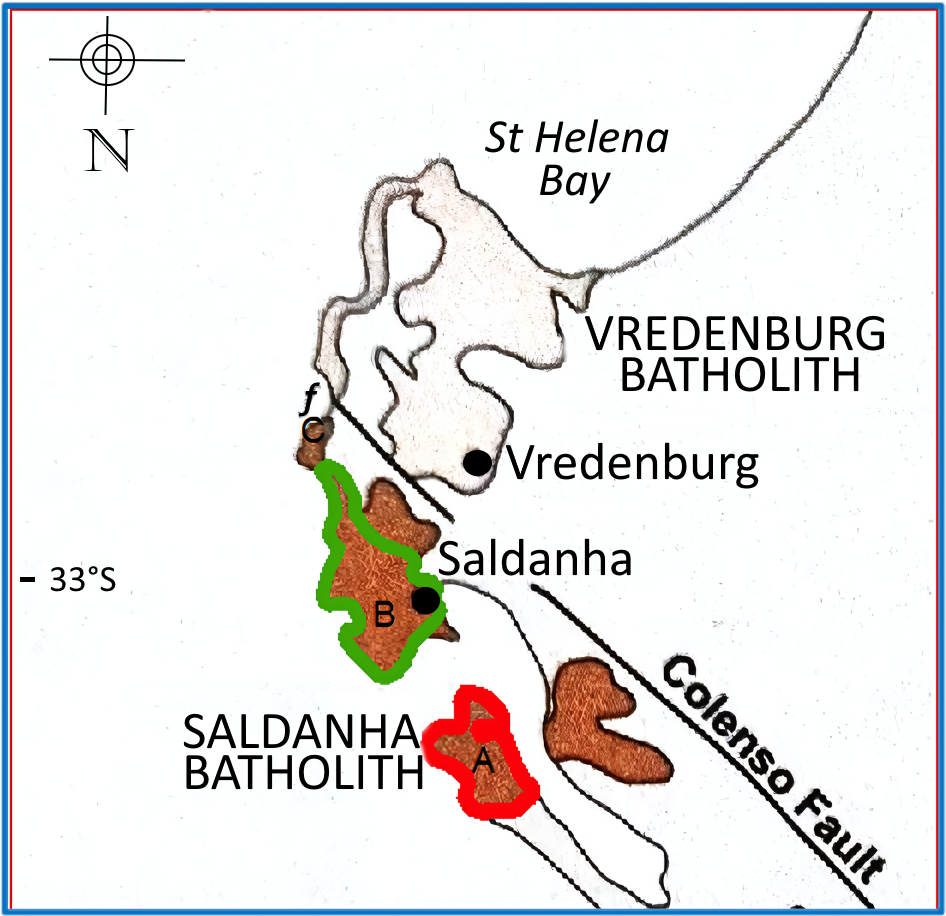
A: Postberg centre including the Tsaarsbank and Plankiesbaai ignimbrites) as well as to the north of Saldanha Bay
outlined in green: –
B: Saldanha Bay and Jacobs Bay ignimbrites) (Clemens and Stevens (2016)) as well as the hypabyssal rocks north of Saldanha Bay. (Map modified from Scheepers and Schoch 2006).
Schoch (1962) in his detailed description of the “cataclasites” at Little North West Bay used the term “rhyolite porphyry” and the symbol V for volcanic as was common on maps of the time to describe the rocks directly to the north east of Little North West Bay (Fig. 1a – location C). These rocks and the related “cataclasites” are also discussed below.
Scheepers (1982) and Scheepers and Cuney (1990) described the breccia pipes on the farm Alexanderfontein in the Darling area (Fig. 1a – location D) and mentioned the presence of foreign “rhyolitic” xenoliths in the breccia pipes. The breccia pipes were interpreted as the root structures of an extensive explosive event open and degassing to surface (by CO2 overpressure). Scheepers (1982) interpreted the “Wolwefontein quartz porphyry” (in close proximity to the breccia pipes) as a subvolcanic feature with miarolitic cavities indicative of a low pressure crystallization event. Isotope analyses of sulphides (molybdenite) in these rocks hinted towards a contribution of source material from the Namaqua – Natal belt. Recent work suggests that the Malmesbury Group are the prime source rocks for the CGS and related rocks. If so, Namaqua – Natal belt material contributed to the Malmesbury Group.
Although the tourmaline matrix supported breccias in the Yzerfontein diorite (Jordaan 1990) has recently been ascribed to “frictional movement during shearing” (Wilson et al. 2023) our proposal is that these breccias are the result of B metasomatism related to volcanism of felsic rocks at a higher level. The Yzerfontein “diorite” is in fact intruded by later phases of quartz syenite. Evidence of B metasomatism is also found in the ignimbrites north of Saldanha Bay (Fig. 1a). It is proposed that circular features in ignimbrite emanated (Fig. 2) from fluid movement along a central conduit and is characterized by an increase in Na as well as Sn, W and B (This theory needs to be investigated in more detail and in particular the locality as described in Fig. 2). Breccias related to Sn mineralization in the Kuils River as well as Somerset West plutons (Schapenberg) are closely related to B-Na-metasomatic events (Rozendaal and Bruwer 1995). Some granites of the Kuils River – Somerset West plutons show clear evidence of subsurface devolatilization.
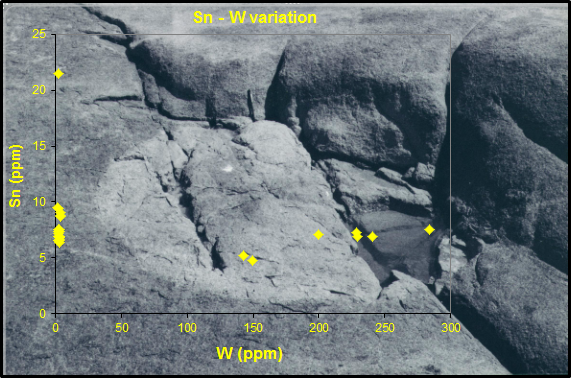
To date, much emphasis has been placed on the volcanic and subvolcanic rocks of the so called Tygerberg terrane (Fig. 1), dominated by peraluminous granites and their volcanic counterparts. It is however, evident from the work of Otto (1957) and Scheepers and Smit (1995), that hypabyssal metaluminous, most likely subvolcanic feeder dyke rocks, occur in the Swartland terrane to the north of the Colenso fault zone (Fig.1). Granite porphyry dykes have also been recorded in the Franschoek tunnel as well as in the Paarl – Klapmuts area. Although the contribution of these dykes to a volcanic magma chamber may not have been proved, Petford et. al. (1994) argued that dykes of this nature (2 m to 20 m in width) are major contributors to the transport of felsic magma in the crust. In the case of the Cape St. Martin dyke rocks their metaluminous nature contrasts with the presence of tourmaline (B) in the dykes. It is however well known that, and in fact illustrated by Scheepers and Smit (1995), fractionation of REE and other trace elements takes place in dykes of this nature. The presence of B reduces the viscosity of the melt and assists in the vertical and lateral transport of magma to subterranean magma chambers that may evolve into volcanic phases (see comments on the recently analyzed metaluminous boulders from Trekoskraal below). The presence of B is also evidence of a change in chemical composition of the feeder magma transported along the dyke over time. This process may have played a role in the transportation of some of the volcanic components related to the Postberg – Saldanha ignimbrites (see B metasomatism above).
Discussion on some Previous results on the CGS volcanics.
Scheepers and Nortjè (2000) announced the existence of a volcanic component related to the Cape Granite Suite and published some of the general characteristics of specific phases of the ignimbrites, particularly in the Postberg area (Fig.1). The chemistry of the Postberg ignimbrites is compared to the chemistry of the “Saldanha quartz porphyry” north of Saldanha Bay and shown to be distinctly different.
The Postberg ignimbrites are further characterized Scheepers and Poujol (2002) and proven to be of Cape Granite Suite age with an age of 515 ± 3 Ma determined on a specific ignimbrite unit. This data clearly denounced the notion that the volcanics are related to events younger than the CGS. The Scheepers and Poujol (2002) age is currently interpreted by some workers to most likely represent a Pb-loss age and an age of ± 540 Ma is currently “accepted” as the age for the volcanic event of the Saldanha Volcanic Complex including the Postberg as well as Saldanha Bay and Jacobs Bay ignimbrite. This age is popular as it neatly represents the age of the end of the major phase of peraluminous granites of the CGS. However, in this discussion the acceptance of this age is reluctant as field evidence to support this age is sparce and in some instances contradictory to current interpretations. Furthermore, the ± 515 Ma age crops up in numerous determinations on the CGS zircons.
Work conducted during the period 2002 to 2006 and published by Scheepers and Schoch (2006) in the textbook on South African Geology supported the fact that the “Saldanha quartz porphyry” (Fig.1) is also part of the volcanic events related to the Cape Granite Suite with most likely the same age as the Postberg ignimbrite. The authors recognized the complex nature of volcanic and subvolcanic rocks within and to the north of Saldanha Bay. They point out that hypabyssal as well as volcanic components are encountered north of Saldanha Bay. Of particular interest is the presence of tuffisite veins indicating a complex transitional regime between hypabyssal, country rock and volcanic units.
In the work of J.D. Clemens; G Stevens (2016) the authors examined field relations and thin-section petrography of the rocks known as the Saldanha quartz porphyry (and indicated as such on the 1:125 000 Geological map of the Saldanha Bay area) and also concluded that the entire sequence, on the northern side of Saldanha Bay, is pyroclastic in character. They proposed that the area around Saldanha Bay is a large volcanic complex consisting of the Postberg and Saldanha eruption centres. They suggest the name Saldanha Bay Volcanic Complex. They defined the low-Ti Saldanha Ignimbrite and the high-Ti Jacobs Bay Ignimbrite as defining two chemically distinct series in the Saldanha Bay area and the more felsic and chemically distinctive Plankiesbaai and Tsaarsbank Ignimbrites composing the bulk of the Postberg ignimbrites.
Clemens, J. D. ; Stevens, G. ; Frei, D. ; Joseph, C. S. A. (2017) made a significant contribution in presenting isotopic and other geochemical evidence that “no apparent magma mixing trends relate the Saldanha to the Jacob’s Bay Ignimbrites, or either of these to the magmas that formed the Plankiesbaai or Tsaarsbank Ignimbrites in the neighbouring Postberg eruption centre”. They propose that “the causes of the cryptic chemical variation among the ignimbrite magmas of the Saldanha centre are variable, but dominated by the compositions of the parent melts and peritectic assemblage entrainment”. This led them to conclude that the common interpretation of silicic calderas as having been formed in large magma reservoirs, with magma compositions shaped by magma mingling, mixing and fractional crystallization, may not be the case. Instead they propose a “more intimate connection between source and erupted magma, and perhaps indicate that silicic magmas are too viscous to be significantly modified by magma-chamber processes”.
Granites and felsic volcanic rocks are commonly associated in space and time (Wyborn and Chappell 1986). It is well known that in many instances the intrusives and the adjacent volcanics are comagmatic (Hildreth 1981), but in other instances (Atherton et al. 1979) the link cannot be established. In both scenarios the investigation of the plutonic vs. volcanic phases contribute significantly to the understanding of felsic magmatism in a specific area. We have no doubt that on the scale of the Saldanha Bay and the Jacobs Bay ignimbrites the relationship between the source and the magma may be intimate, but on the scale of the eruption sequences within each of these, chemical variation in the magma chamber over eruption time undoubtedly played a role. This is clearly evident in the Postberg area and the variation in chemistry of the Postberg ignimbrites as well as in the Saldanha Bay and Jacobs Bay ignimbrites (Fig. 3 and Fig. 4).
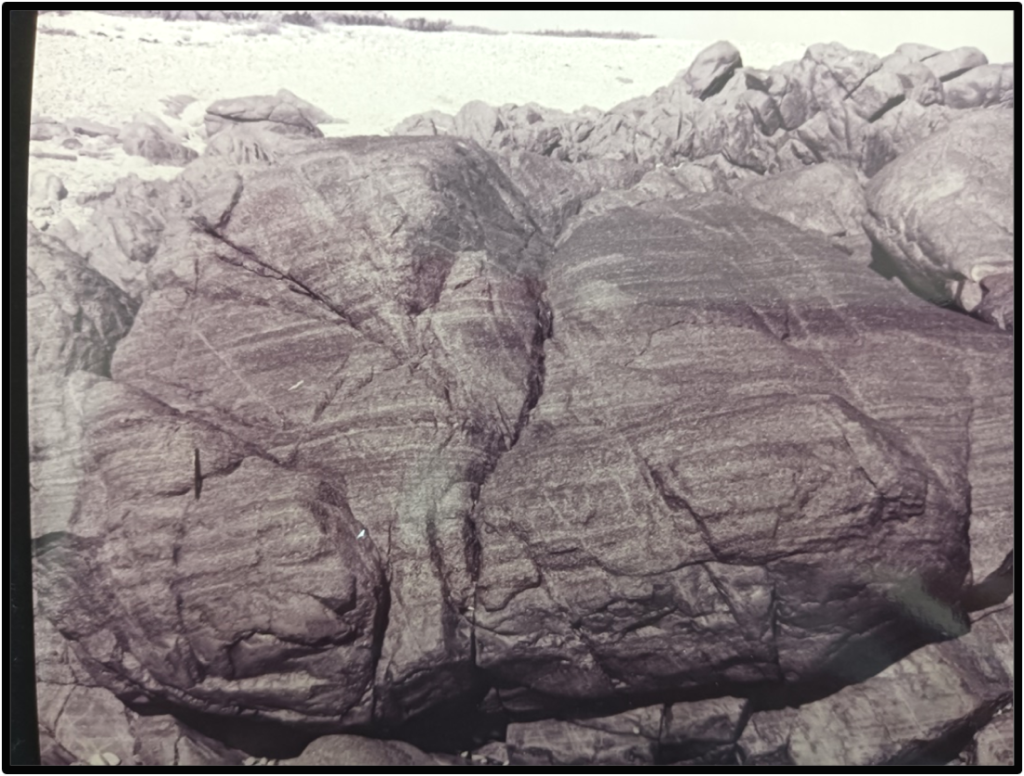
In his M.Sc. thesis Misrole M (2020) made a significant contribution to the geochemical data of the various ignimbrites. The author provides new geochronological data that seem to suggest that the S-type volcanics of both the Postberg and Saldanha Bay volcanic centres are part of the peraluminous phase (Phase 1) of the CGS as defined by Scheepers (1995) and in fact signifies the end of the peraluminous phase of magmatism of the CGS at around 538 to 540 Ma. Although this would in general comply with the evolutionary trend of granitic magmatism over time related to subduction, it may not be applicable to the CGS. Scheepers (2000) suggested that provision of CGS granites and volcanic melts may be attributed to a single heat event with:
- early commencement of peraluminous melt and crystallization of plutonic rocks, intrusion of metaluminous melt and crystallization of plutonic rocks followed by intrusion of I type and eventually A-type granites under maximum heat supply conditions.
- although the above scenario implies peraluminous melts (S-type granites and volcanics) to have been depleted prior to commencement of I-type granitoids crystallization, this would be an extremely simplified interpretation in the case of the CGS. If A-type granites are indeed young (assumed 518 Ma?) it would be highly unlikely that the high-level intrusion of these high temperature melts would not initiate a renewed peraluminous producing melting phase in the Malmesbury rocks. Particularly during the onset of tensional tectonics. Hence the potential presence of younger volcanic peraluminous phases.
J.D. Clemens; P.M. Marara; G. Stevens; J. Taylor (2022) mention that the Saldanha eruption centre has an age of ± 542 Ma. The basal Saldanha Ignimbrite is partly intermingled with and partly overlain by the Jacobs Bay Ignimbrite, both having S-type characteristics. In the lowermost parts of the Jacob’s Bay Ignimbrite they identified, amongst others, non-fragmental, hypabyssal rocks that were evidently intruded prior to (thus OLDER than) the explosive intracaldera eruptions that formed the Saldanha ignimbrites. The following is paraphrased from the Clemens et al. (2022) abstract: “Beach cobbles and pebbles, sampled from the Trekoskraal coastal area, include three texturally and chemically distinct groups – rhyolitic ignimbrites, rhyolitic hypabyssal rocks and dacitic hypabyssal rocks or lavas. Only a minority of these rocks (from the rhyolitic ignimbrite group) show some chemical affinities with the Saldanha Bay ignimbrites. The other pebble types show neither chemical nor textural similarities with the rocks of either the Saldanha or the Postberg eruption centres. The pebbles and cobbles also have no chemical affinities with any of the granitic intrusive rocks of the region. Their chemical and isotopic characteristics suggest that a variety of different magma batches were formed through partial melting of heterogeneous Malmesbury Group metamorphic rocks, at depth. LA-ICP-MS dating of igneous zircon crystals from two of the pebbles (a low-silica rhyolite ignimbrite and a dacite) yielded magmatic ages of 540 ± 4 Ma and 533 ± 4 Ma, respectively. Taking uncertainty brackets into account, these new dates suggest that there may have been a 3 Myr hiatus in eruptive activity, between the eruptions responsible for the exposed Saldanha ignimbrites and the eruptions that produced the volcanic units from which the pebbles were derived. This confirms the inference that there was a previously unidentified, later (YOUNGER), volcanic event associated with the Cape Granite Suite in the Saldanha area”.
The fact that older (than the ignimbrites) hypabyssal rocks are present in the Saldanha area is a known fact (west of the Colenso fault zone). In addition, examples of hypabyssal rocks (such as the Cape St. Martin dyke rocks) are found to the east of the Colenso fault as described above (most likely younger or the same age than the ignimbrites of the Saldanha area). The fact that the various sea levels known on the West Coast of up to 90 m above current mean sea level may have sourced boulders (pebbles) from an extensive geographical area, a wide variety and locality of sources renders interpretations on the nature of the pebbles immature. In addition:
- 540 ± 4 Ma = 536 – 544 Ma. These ages very much overlaps the “accepted” age of the Saldanha eruption centre.
- 533 ± 4 Ma = 537 – 529 Ma. Also overlaps many of the “accepted” ages of the volcanics (Misrole 2020).
Although the authors of this discussion favor the interpretation that younger (than ±540) volcanic events are part of the CGS history, this publication (Clemens et al. 2022) unfortunately does not provide convincing evidence. The ages as provided in fact confirms nothing about the existence of a later (younger) volcanic event based on the data provided, even if the boulders/pebbles are in fact part of the CGS volcanic event. It in fact confirms that the ages presented by various publications needs to be interpreted very carefully.
Some aspects to be addressed.
Intracaldera Ignimbrites
Ignimbrite deposits are known to cover large areas of countryside on the flanks of calderas or further away, covering large areas in voluminous masses.
The fact that the Postberg ignimbrites are directly overlying Malmesbury Group rocks and in places contain xenoliths of Malmesbury rock, in our opinion opens the possibility that we are most likely not looking at intracaldera ignimbrites (at least in the Postberg area) but at flows away from the caldera, at least in certain localities (if calderas in fact exist in the area). If the interpretation of Clemens and Stevens (2016) that the ignimbrite/Hoedjiespunt contact on the Danger Bay shoreline represents an erosion surface of the Hoedjiespunt granite covered by an ignimbrite flow is correct, the intracaldera nature of the ignimbrite requires detailed explanation. In close proximity to the supposed “erosion contact” Malmesbury xenoliths are found within the ignimbrite or hybabyssal rock. If the Hoedjiespunt granite surface was exposed, Malmesbury on top must have been eroded unless the volcanism was coincidentally in the vicinity of the Malmesbury / Hoedjiespunt contact. A very likely scenario is that horst and graben features in the Saldanha Bay and Postberg areas are predominantly controlling geological feaures at a.m.s.l. (average mean sea level) as seen today and that an interpretation of the intracaldera nature of the ignimbrite may be applicable in some places but not others.
The possibility that the ignimbrite flows are predominantly controlled by activity along the Colenso fault zone over time should be investigated in detail. Central calderas may not even be playing a role but ignimbrite eruptions that were controlled by degassing along the Colenso fault zone over an extended period of geological history. Evidence of this is provided by the Massenberg ignimbrite? and the fact that vertical displacement seems to control the granite and volcanics current positions. The conclusion that peraluminous magmatism ended at the end of Phase 1 of the Scheepers (1995) CGS event (Misrole 2020) may then also be doubted. The presence of peraluminous magma over a longer time period (even as young as 515 Ma) is then explained simply be the ability of peraluminous melts to surface along fractures (thus controlled by a mechanical process) along the Colenso fault zone.
Age relationships.
If the 542±2 Ma age of the ignimbrite (Clemens and Stevens 2017, Misrole 2020) is accepted, some explanation of the field relationships of CGS granites in the Saldanha area are required.
The accepted age of the Trekoskraal granite is 539 ± 4 Ma (Scheepers and Armstrong 2002). This age clearly overlaps the 542 ± 2 Ma age of the ignimbrite implying that an ignimbritic volcanic event coincided with the crystallization age for the Trekoskraal granite (and the Seeberg granite at 539,7 ± 3,6 Ma (Scheepers and Armstrong 2002)) for that matter. The age of the Langebaan granite porphyry, a rock with textural evidence of devolatilization is 541.1 ± 2.7 Ma (Unpublished data Scheepers and Armstrong 2002). As explained below the simultaneous emplacement of Trekoskraal granite and ignimbrite presents a problem. The cataclastic deformation of the Trekoskraal granite, as currently explained, must have happened over a long time period. The Trekoskraal granite needed to cool down under conditions suitable for brittle deformation, then be cataclastically deformed extensively over a long time period. To have volcanics at the same level with the same age is a practical problem that needs to be explained.
Ignimbrite, Trekoskraal granite, Seeberg granite and Langebaan granite porphyry rocks outcrop at the same level (for practical purposes). Although vertical displacement along NW-SE (dominant) and NE-SW shearing and faulting may be invoked to explain the current level and equivalent ages of intrusives and extrusives, some other possibilities may be explored:
a) Intrusive and volcanic events as well as deformation of plutonic rocks took place over a short time span of ±2 Ma.
b) Scheepers and Armstrong (2002) concluded an age of 552,1 ± 3,9 Ma (95% confidence) for sample HPT-1, a biotite rich variety of the Hoedjiespunt granite. Calculation of a concordia age on 5 of the data points from sample HPT-8 (biotite poor variety of the Hoedjiespunt granite) yielded 543 ± 10 Ma (95% confidence). Granted the large error on the determination, it is of importance to note the 543 Ma age, similar to the “accepted” ages of the ignimbrites. In essence this age suggests that within the same (Hoedjiespunt) magma, zircons display an age variation, possibly controlled by the variation in the peraluminicity of the magma batches.
c) Peraluminous zircons are notoriously complex. The work of Scheepers and Armstrong (2002) was based on:
- Large samples sizes of >50 kg and separation of numerous populations of zircons from each sample.
- Cathodoluminescense work of weeks per sample selecting zircons on which the rims (last crystallization phase) was clearly visible and using extremely small spot sizes to analyze these rims.
d) The above approach clearly outlined:
- The last phase of crystallization (rims) were the only region on the zircon representing the last crystallization phase (age) of the magma. A huge variety of ages were represented by the various inner (inherited) parts of which 1655 Ma and 928 Ma dominated but age groups around 720 Ma and 790 Ma were also encountered. The same could be said for the Trekoskraal and Seeberg granites (±539 Ma).
- The coincidence in ages of the biotite poor Hoedjiespunt granite, Trekoskraal granite, Seeberg granite, Langebaan granite and the various “accepted” ignimbrite ages needs to be explained. Surely the Hoedjiespunt granite age, for instance, must raise red flags on the granite being an erosion surface on which ignimbrites were deposited as well as showing ages as low as 543 Ma. Granted, 10 Ma may be ample time to erode Malmesbury as well as Hoedjiespunt granite to a level where ignimbrite flows may cover the granite. Leygonie (1977) concluded, based on field evidence, that the Hoedjiespunt and Seeberg granites are in fact the same granite with textural and mineralogical variations over outcrop area. This would explain the poorly constrained ±543 Ma age obtained from the biotite poor Hoedjiespunt granite. The dominance of granites with a ±540 age in the Saldanha-Langebaan area may open the possibility that the ±552 Ma age of Scheepers and Armstrong (2002) may represent preserved batches of Hoedjiespunt granite with early (old) crystallization of zircon from highly peraluminous batches in the melt. Xenoliths (unclear whether they represent autoliths) of granite do occur in the Hoedjiespunt granite with resorption textures that attests to the complex nature and history of the Hoedjiespunt granite.
e) Images published by Misrole (2020) (as an example) on spot positions and sizes on zircons from the various ignimbrites on which the ±540 Ma events were concluded as a fact, raises serious doubts on what these ages represents taking the above into consideration.
f) Scheepers et al. (1999) outlined the difference in zircon chemistry from S-, I- and A-type CGS rocks. S-type zircons in particular are showing major chemical variation from one growth cycle to the next illustrating changes in magma composition and chemistry and thus complexity in magma batches.
g) In light of the large variation in chemistry of the various ignimbrites as published by various authors (SiO2 69 – 78 wt%; MgO 14.5 – 25 wt%; TiO2 0,15 – 0,51 wt%; Zr 100 – 400 ppm) it is clear that the volcanic/subvolcanic phase of the CGS is complex. In contrast to the conclusion of Clemens et. al. (2017), that magma chamber process might play a subsidiary role to the relationship between source and characteristics of the erupted magma, field evidence seem to suggest the contrary. At least, there is a clear difference between the Jacobs Bay “ignimbrites”, their chemistry and field relationships and the remainder of the ignimbrites. Field evidence provides clear evidence of vastly different characteristics of erupted magma over short distances in space (Fig. 3 and Fig. 4) and most likely time. Numerous examples of these features are encountered on both side of Saldanha Bay.
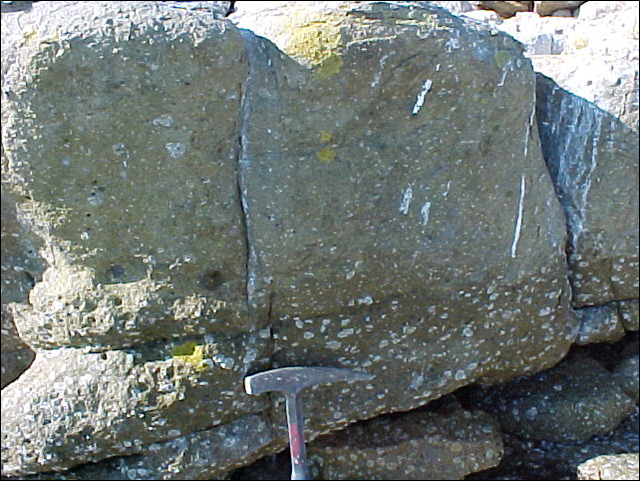
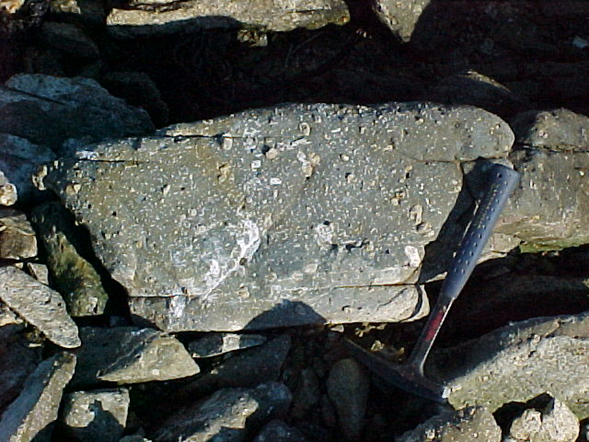
The possibility should be considered that the “accepted” age of ± 540 Ma for the ignimbrites simply represent the age of inherited zircons (the Trekoskraal, Seeberg and Langebaan granites) assimilated in the ignimbrite phase magma chamber/s and erupted over time along the Colenso fault zone during subsequent and long lasting activity on the fault zone. Further evidence for this is the presence of the Massenberg ignimbrite?, away from the Saldanha Bay volcanic event area, but in close proximity to the Colenso fault. The “Colenso fault zone” has been proven (unpublished gravimetric and magnetic data (Scheepers and Ameglio 2005)) to consist of a shear zone with numerous individually definable shear zones over a distance of 18 km (width) trending in a NW direction. In essence, it is proposed that peraluminous melts are sourced over time by movement along these shear zones. Cycles are short (thousands of years to tens of thousands of years), medium (millions of years) and long (tens of millions of years). This would contradict the proposal of Scheepers and Nortjé (2000) and subsequent workers that the ignimbrites are related to one or more “central calderas”.
It would be highly coincidental if the several phases of plutonic rocks on the Tygerberg terrane (Western) side of the Colenso fault (at least 8 individually identifiable plutonic rocks, each with distinct chemical compositions and textural features, as well as at least four ignimbrite phases (with distinct chemistries), numerous hypabyssal phases, all with ages around 540 Ma, did not have a shared genetic component. IF these rock were all generated by melting of Malmesbury metasediment, the following is suggested:
Volatile rich and volatile poor batches of magma are generated by Malmesbury sediment melting, most likely by a heat event as result of intrusion of high K calcalkaline magma. ii) Hoedjiespunt/Seeberg felsic magma is transported to surface by early movement along the Colenso fault zone. iii) Early high temperature batches of this magma are high in zirconium, zirconium remains in melt and early crystallization (552 Ma) of new zircon layers (covering inherited zircon) takes place in peraluminous melt batches (high biotite concentration) (coinciding with the crystallization of pluton rim phases of Hoedjiespunt granite, now observed as autoliths). iv) New batches of magma mingle with the early Hoedjiespunt batches and Seeberg and Trekoskraal granite crystallization takes place. Intensification of movement along the Colenso fault over time is observed by rounded phenocrysts in the Trekoskraal granite. v) Increasing volatilization of magma batches along individual fractures of the Colenso shear zone takes place. Further crystallization of zircon layers on rims of early (542 Ma) crystallized zircon is prohibited by decrease in peraluminicity of the magma as well as an increase in vapour pressure. vi) Volatile rich silicic magma is transported to higher levels in the crust as distinct separate batches inheriting 542 Ma zircon crystals from earlier events. vii) Rapid eruption of magma by propagation of melt under brittle crustal conditions (see discussion of cataclasites below) prohibits the crystallization of zirconium on already crystallized 542 Ma zircons.
The Trekoskraal “Cataclasites”.
Previous research on the cataclasites at Duminy Point, south of North West Bay, bordering the farm Trekoskraal in the Vredenburg district, focused on the mechanical deformation of the Trekoskraal granite supposedly by movement on the Colenso fault zone bordering the area of interest to the north (see Schoch 1961 and Kisters et al 2002 etc.) (Fig. 1).
Recent work (in progress) investigates:
a) the age relationship between the Trekoskraal granite(s) and the ignimbrites.
b) the assumption that the current status of the rocks is the result of simple dextral and sinistral shear as previously proposed. We are of the opinion that at least some of the deformational features observed in the Trekoskraal granite are the result of violent catastrophic brittle shattering due to the extrusion and intrusion features related to the Postberg ignimbrite and “Saldanha Complex” ignimbrites. Protomylonite, ultramylonites, pseudotachalites, flinty chert rock etc. are all terms that commonly associated with calderas and ignimbrite formation on or in close proximity to strike slip faults or normal slip fault. This is evidently the case for the “Saldanha Complex” ignimbrites. Fault and shear zones are most likely dominantly related to movement on the Colenso fault zone, but also younger events. Horst and Grabens undoubtedly complicates interpretation of the geological features
Close examination of the Trekoskraal cataclastic rock reveal textural features (Fig. 5) that may possibly be interpreted as evidence of catastrophic cataclastic shattering due to the ignimbrite event. Melt features would in this case be related to ignimbrite melt violently introduced into wall rock of Trekoskraal granite (tuffisite). It is suggested that several episodes of “magma” injection and brittle deformation occurred during the duration of the volcanic event.
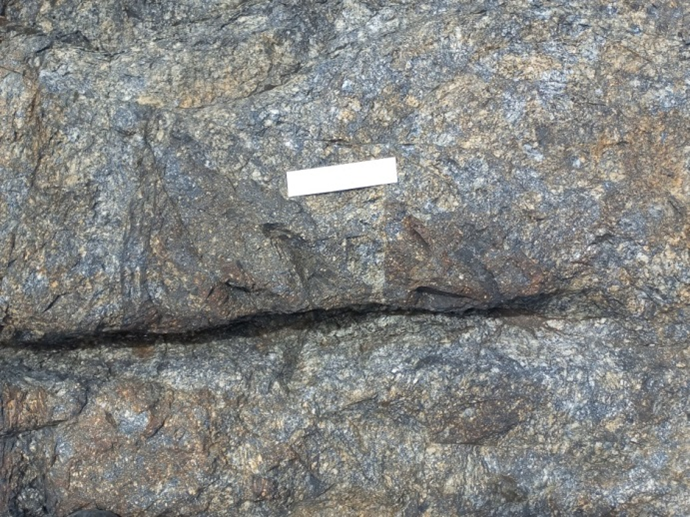
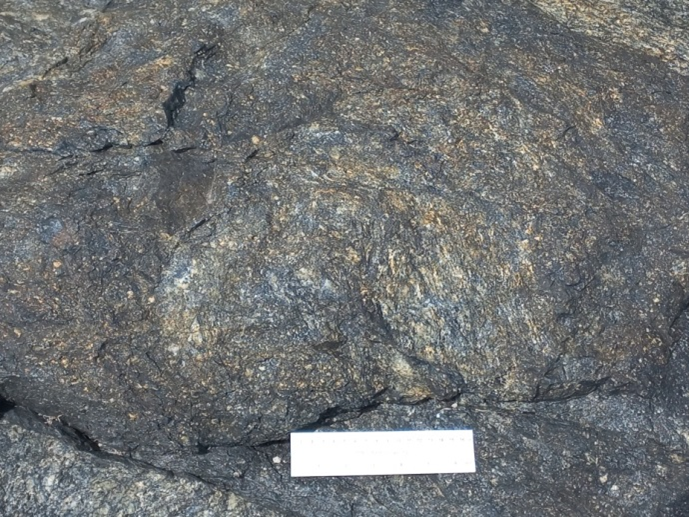
Examples of cataclasticly deformed granite with rounded phenocrysts (such as the Trekoskraal granite) are commonly found in both the Postberg and Saldanha Bay ignimbrites (Fig. 6). These deformed granite xenoliths provides ample and widespread evidence that brittle deformation of granitic rocks took place over an extended time period prior to the intrusion of VARIOUS ignimbrite events. “Cataclasites” presented in Fig. 6 b and c were sampled approximately 18 km to the west of what is interpreted as the main Colenso fault zone, illustrating that ignimbrite intrusion are very closely linked to “cataclasite” formation.
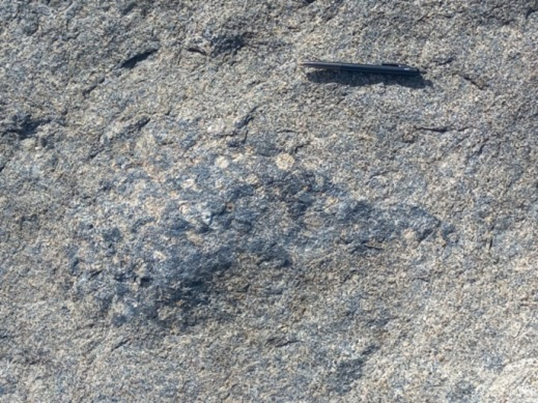
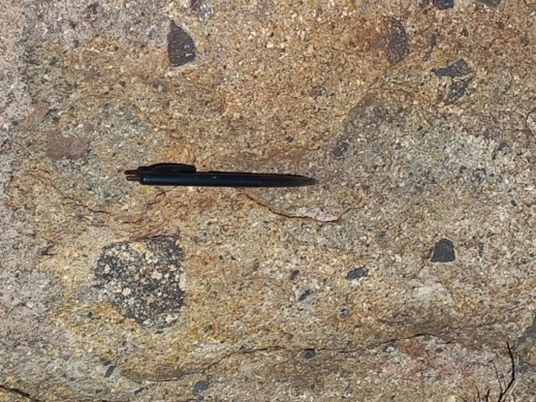
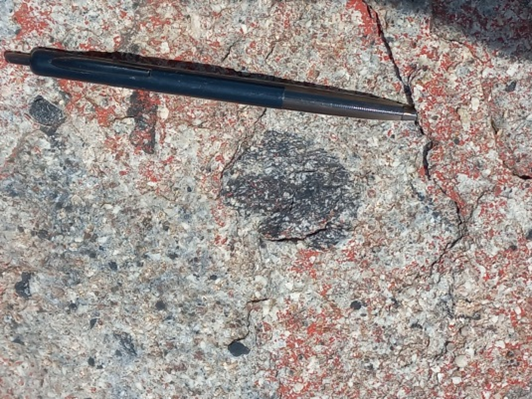
In summary, it is evident that:
- The Trekoskraal granite at Trekoskraal has an age of 539 ± 4 Ma.
- The “accepted” age of the ignimbrites appears to be 542 ± 2 Ma.
- The above two ages clearly overlap.
- The Trekoskraal granite show brittle deformation, most likely as a result of deformation during melt advancement, catastrophic failure and melt injection as well as strike-slip brittle deformation.
- The evidence of d) taking place is widespread over the entire Saldanha Bay ignimbrites as well as the Postberg ignimbrites attesting to the fact that granites such as the Trekoskraal granite, the Seeberg granite and the Langebaan granite porphyry (to a lesser extent) (all within the ±540 Ma age bracket) were already cooled down to react under brittle deformation conditions OVER AN EXTENDED TIME PERIOD.
- If strike-slip deformation is accepted as the only mechanism of producing cataclasites (and pseudotachyllite), the problem with the ignimbrite age also being in the ±540 Ma bracket is even more complicated (evidence of strike-slip deformation over an extended time period).
Some facts to consider:
- Cataclastic deformation of the Trekoskraal granite and/or shattering of the Trekoskraal granite by the ignimbrite predates the intrusion of the ignimbrite as cataclasites/shattered fragments are found as xenoliths in the Postberg ignimbrite (If most or all of the fragements are indeed Trekoskraal granite fragments).
- If the above is correct then the following needs to be considered:
a) The Postberg ignimbrite is significantly younger than 539 ± 4 Ma (515 ± 2 Ma as published by Scheepers and Poujol ? – admittedly a poorly constrained date). Recent dating suggests ± 538 Ma (Misrole 2020) for both the Postberg/Tsaarsbank centre and the Saldanha/Jacobs Bay centre. This would place the ignimbrite as overlapping with the Trekoskraal granite.
b) The fragments of rounded phenocryst and cataclastically deformed/shattered granite as xenoliths in the Postberg ignimbrite may not Trekoskraal granite. The degree of deformation of the fragments however, corresponds with in situ observations of the Trekoskraal granite at the type locality. Previous studies inferred a extended history of dextral and sinistral shearing of the Trekoskraal granite, not consistent with a short time span that would explain the intrusion of the granite, cooling, deformation and emplacement of the ignimbrite. If the cataclasites are of various granites it would confirm the close genetic relationship between ignimbrites and shearing.
- Evidence for numerous ignimbrite eruption episodes is abundant. Recrystallized ignimbrite fragments are enclosed in younger flows, xenoliths of a range of various granite types are characteristic of ignimbrite flows of different episodes.
- The Tsaarsbank/Postberg ignimbrite has a third component on the southwestern edge of the Langebaan lagune.
Priorities.
The above discussion highlights the need for:
- thorough and detailed mapping of the Saldanha Bay area and surroundings.
- combining geological mapping with geophysical measurements (magnetic/radiometric/AMS).
- Use the results of mapping and geophysics to conduct a carefully controlled sample exercise to interpret the geochemistry and geochronology of plutonic and volcanic rocks of the Saldanha Bay area.
d) Testing the following hypotheses:
i) The Trekoskraal granitic magma intruded along the Colenso shear zone.
ii) Feldspar pehenocrysts in the magma show features of Rapakivi textures and were most likely partly rounded by mechanical abrasion.
iii) The uppermost part of the Trekoskraal magma were solidified and to an extent affected by dextral/sinistral shearing related to the Colenso fault zone when the first of the “New Saldanha Complex” ignimbrites erupted.
iv) Ignimbrite melt advancement and eventually explosion violently forced ignimbrite melt in to the Trekoskraal granite, shattering the rock, incorporating portions of Trekoskraal granite in the melt and creating a network of melt-filled fractures through the Trekoskraal granite. This would imply that the ignimbrite event is significantly younger than early Trekoskraal granite.
v) In places (see V marked on Schoch 1961 map and described as rhyolite) ignimbrite melt incorporated early crystallized ignimbrite or “rhyolite” and may be recognized as such.
vi) The ignimbrite event took place repeatedly over an extended time period.
vii) Dextral and sinistral movement on the Colenso fault zone overprinted the ignimbrite shattering event and in places followed existing weak zones.
REFERENCES.
Atherton, M. P., McCourt, W. J., Sanderson, L. M. & Taylor, W. P. in Origin of Granite Batholiths: Geochemical Evidence (eds Atherton, M. P. & Tarney, J.) 45–64 (Shiva, New York, 1979).
Clemens J.D. ; G Stevens.(2016). The Saldanha Bay Volcanic Complex: Clarifying the Cambrian geology of the Postberg – Saldanha Area, West Coast, South Africa. SouthAfrican Journal of Geology (2016) 119 (2): 347–358.
Clemens, J. D. ; Stevens, G. ; Frei, D. ; Joseph, C. S. A. (2017). Origins of cryptic variation in the Ediacaran-Fortunian rhyolitic ignimbrites of the Saldanha Bay Volcanic Complex, Western Cape, South Africa. Contributions to Mineralogy and Petrology, Volume 172, Issue 11, article id.99, 23 pp. Pub Date: December 2017.
Clemens J.D.; P.M. Marara G. Stevens J. Taylor (2022). Magmatic clasts in the Saldanha ignimbrites, and Trekoskraal beach pebbles: missing pieces from the volcanic puzzle in the Cape Granite Suite. South African Journal of Geology. Vol. 123, No. 1.
Hildreth W. (1981). Gradients in silicic magma chambers: Implications for lithospheric magmtism. Journal of Geophysical Research (86). Issue B11. Pp. 10153 – 10192
Joseph C.S.A (2013). The petrogenesis of the ignimbrites and quartz porphyritic granites exposed along the coast at Saldahna, South Africa. M.Sc. University of Stellenbosch.
Otto J.D.T. (1957). The dyke rocks of Cape St. Martin. Ann. Univ. Stellenbosch, 33A (8), 387-443.
Misrole M.(2020). A re-assessment of the geochronology and geochemistry of the Postberg ignimbrites, Saldanha, Western Cape, South Africa. A thesis submitted in fulfilment of the requirements for the degree of Magister Scientiae in the Department of Earth Science, University of the Western Cape 123 pp.
Rozendaal A. and L. Bruwer (1995). Tourmaline nodules: indicators of hydrothermal alteration and Sn, Zn (W) mineralization in the Cape Granite Suite, South Africa Journal of African Earth Sciences. Volume 21, Issue 1, July 1995, Pages 141-155
Schoch A.E. (1962). The cataclasites of Northwest Bay. Ann. Univ. Stellenbosch, 37, Ser. A, 659.
Scheepers (1982). Die Uraan-, Thorium- en Molibdeen – verspreiding in Geselekteerde leukograniete van die Kaap- Graniet Suite. M.Sc. tesis. Univ. Stellenbosch.
Scheepers, R. and Nortjé (2000). Volcanism related to the Cape Granite Suite. 27th Earth Science Congress of the Geological Society of South Africa, Stellenbosch. Journal of African Earth Sciences, 2000, 31 (1), 65.
Scheepers R. and C.M. Smit (1995). Fractionation of REE in highly evolved metaluminous felsic dykes. Jnl. Afr. Earth. Sci. 21(1), 71-90.
Scheepers R., Cousin L.E., Przybylowicz W.J.and Prozesky V.M. (1999). Nuclear microprobe (PIXE) Analyses of Zircons as Indicators of Granite Type. Nuclear Inst. and Methods in Physics Research, B. 158. 599 – 605.
Scheepers, R. and Nortjé, A. N., 2000. Rhyolitic Ignimbrites of the Cape Granite Suite, south-western Cape Province, South Africa. Journal of African Earth Sciences, 31,647-656.
Scheepers, R. and Poujol, M., 2002. U-Pb zircon age of Cape Granite Suite ignimbrites: characteristics of the last phases of the Saldanian magmatism. South African Journal
of Geology, 105 (2), 163-178.
Scheepers R. and A.E. Schoch (2006). The Cape Granite Suite. pp. 421 – 432. In: Johnson, M.R., Anhaeusser, C.R. and Thomas, R.J. (Eds.) (2006). The Geology of South Africa. Geological Society of South Africa, Johannesburg/Council for Geoscience, Pretoria, 691 pp. S1687-8507(21)00045-5: 9781919908779.
Wilson S.; R. Bailie; C. Harris; S. Dunn; V. Silinda; S.N. Lembede (2023). The origin, nature and fluid characteristics of the hydrothermal veining crosscutting the Yzerfontein gabbro-diorite, Cape Granite Suite, South Africa. South African Journal of Geology (2023) 126 (3): 275–294.
Wyborn, D. and Chappell, B. W. (1986) The petrogenetic significance of chemically related plutonic and volcanic rocks units. Geol. Mag., 123, 619–628.