What you might gain by reading this:
As a geologist not specializing in gemstone deposits you will gain insight into some fascinating geological events that describe how tanzanite was formed. It is written in a less technical style to simplify the text for casual readers.
As a non-geologist with an interest in tanzanite you will obtain an appreciation for the effort nature made to bring you this unique gemstone – even if some of the terms are a bit obscure!
- WHY IS TANZANITE SO UNIQUE?
The tanzanite (Fig. 1) in your ring, pendant, bracelet, bank vault or gemstone collection is mined in a single 5 km long by 100 m wide strip (roughly the length of Kilimanjaro airport runway), in the Republic of Tanzania (Fig. 2). There is as yet no other source on earth. We will address the rarity of tanzanite and illustrate how many aspects of gemstone formation had to happen at the right place, time, manner and sequence to create this gemstone.
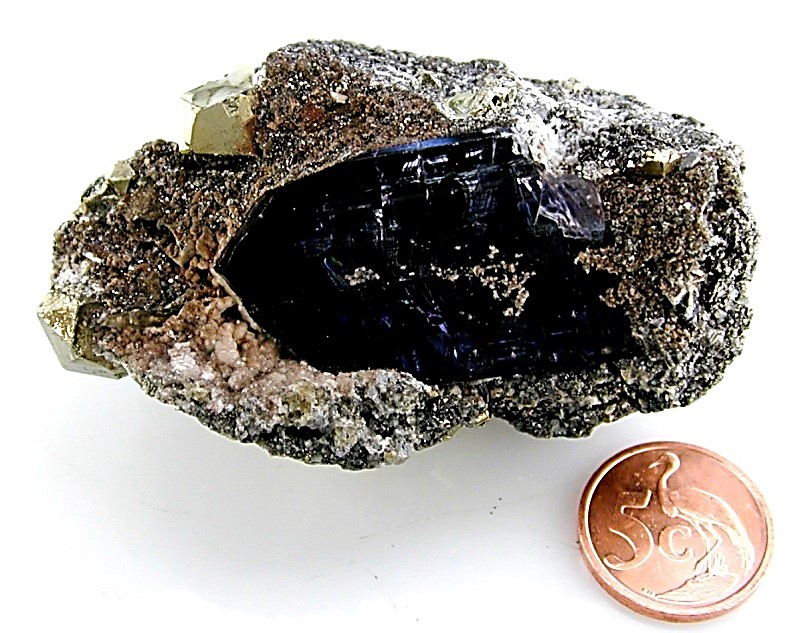
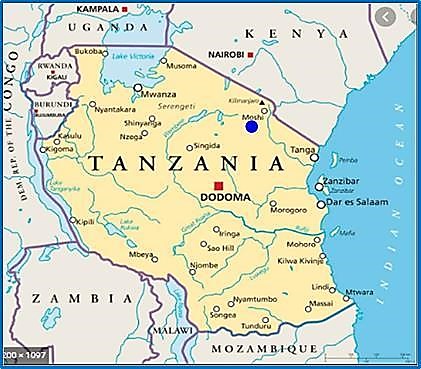
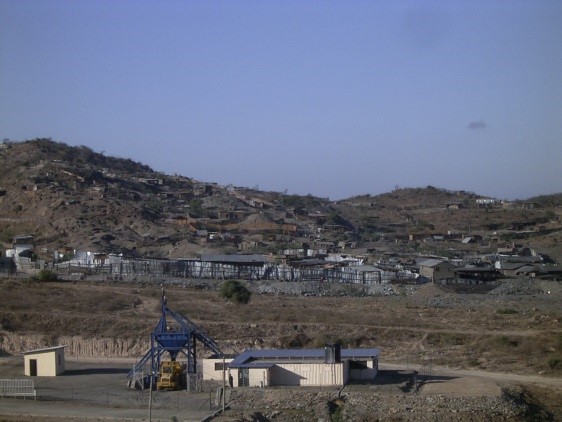
- INTRODUCTION.
To enable us to understand the uniqueness of tanzanite, it is important to recap minerals in general and specifically the silicate or rock forming minerals. Tanzanite is a silicate mineral. The silicates or rock forming minerals are exactly what the name implies – the minerals that form the most common rocks comprising the earth’s mantle and crust (Fig. 3a, b).
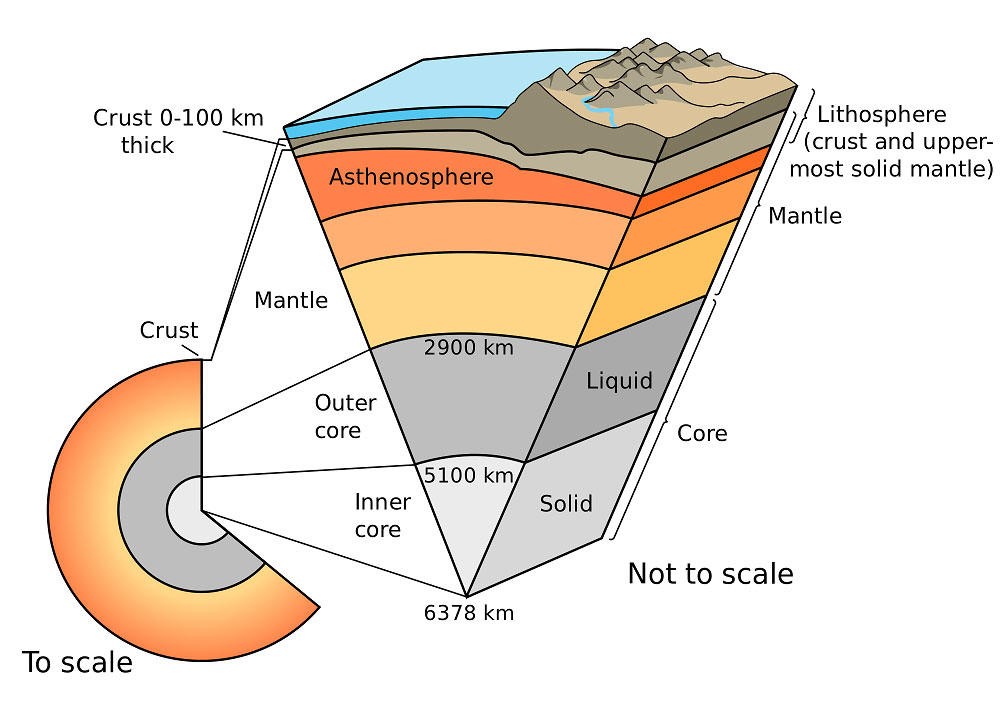
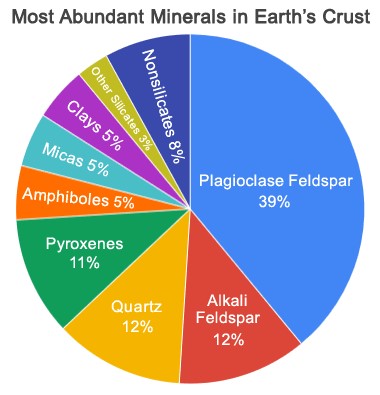
Although there are numerous minerals that are not silicates such as Table Salt (NaCl – A CHLORIDE), Fluorite (CaF2 -A FLUORIDE used in toothpaste), Molybdenite (MoS2 – A SULPHIDE used as a lubricant), Apatite (CaPO4,F,Cl – A PHOSPHATE found in teeth) and Korundum (Al2O3 – AN OXIDE used as an abrasive) many common coloured gemstones such as Amethyst, Emerald, Tourmaline, Tsavorite, Rhodolite and Tanzanite belong to the silicate minerals.
There is thus no evident reason why tanzanite (a silicate) should be a rare gemstone – or is there?
3. WHAT IS TANZANITE?
Tanzanite is a blue coloured variety of Zoisite (Named after Sigmund Zois, a naturalist in 1797). Zoisite is a silicate mineral very common in metamorphic rocks with the general formula of:
Although there are other coloured varieties of the zoisite group as per example green zoisite (Fig. 4)
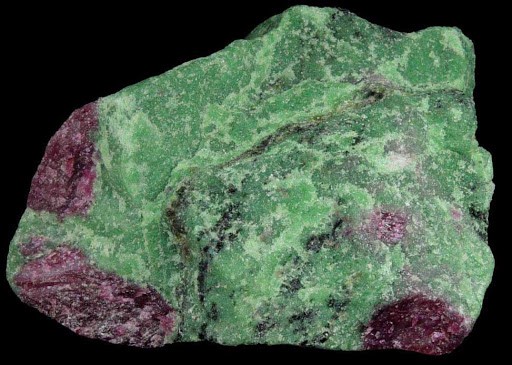
and golden zoisite, common zoisite is colourless or light brown.
Tanzanite in its natural form may be blue/purple, light blue or brown (Fig. 5). Tanzanite, often referred to as the prince of gemstones, is a perfect example of an extremely rare gemstone formed by a series of events taking place over many millions of years. In the next paragraphs we will attempt to convey some of the principles of why there has been, as yet, only one tanzanite deposit discovered on earth. We will also discuss some of the myths regarding the formation of the gemstone as well as some outright wrong beliefs that has become entrenched in the literature
.
If tanzanite is a variety of zoisite, which is a common rock forming mineral, why is it so unique?
The general formula for tanzanite may be written as:
[(Ca V3+Fe3+Al2{SiO4}3{Si2O7}(OH)]
Compared to colorless zoisite, one difference is the presence of V (Vanadium) (and Fe (Iron)). V3+ is the so called chromophore (colour causing element) in the structural formula of tanzanite. Other examples of chromophores are Cr (Chromium) and (V) in Emerald and Mn in Rhodolite (Garnet).
Herein lies the problem: – Tanzanite is formed as an Aluminium (Al) rich mineral with up to 33 wt.% Al2O3. Wherever Al is concentrated in the Earth’s crust, you do not find V in significant quantities. Normal earth processes tend to separate the two elements. As an example we see in Fig. 6 that there are up to 8 weight percent of Al in the Continental crust but less than 0.1 weight percent of common trace elements including V (V crustal concentration is 140 ppm on average in the crust).
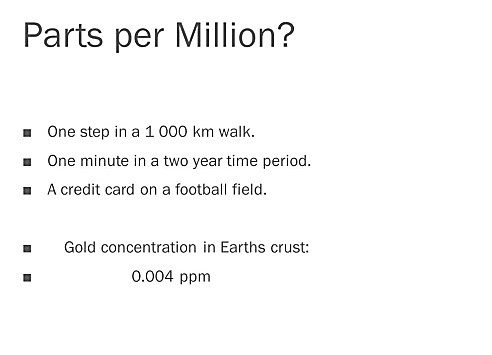
4. WHY IS TANZANITE FORMED WHERE IT IS?
The first reason why tanzanite is so unique is that it occurs in a place on earth where geological processes succeeded in concentrating Al and significant quantities of V in a confined space. Coincidentally, Ti (Titanium), an element also present in tanzanite and playing an important role in its colour, was also present in significant quantities (Fig. 7).
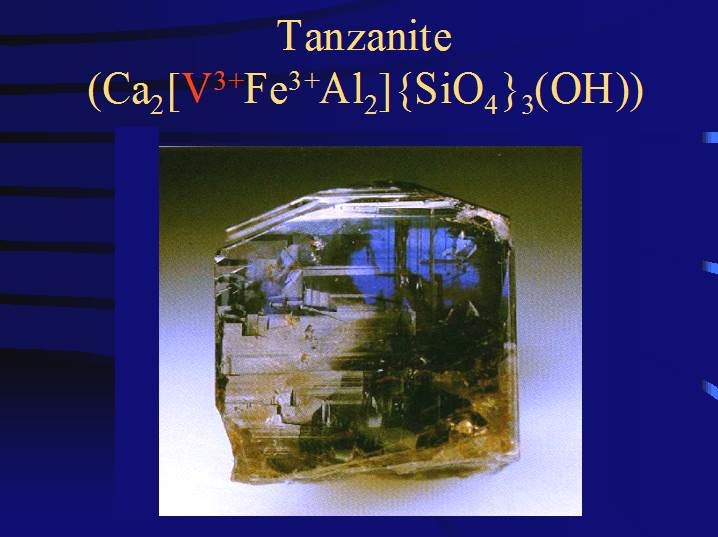
Two concepts that we also need to emphasize are:
- the role of time and
- the fact that Earth is a continuously changing planet, it has undergone extensive evolution in its past and is still continuing to do that.
Without the combination of time and the constant weathering, burial, metamorphism and deformation of rocks, tanzanite would never have existed.
The TIME aspect.
The first steps that eventually led to the formation of tanzanite commenced 1.6 to 1.8 billion years ago when rocks with a significant crustal chemical signature increasingly began to accumulate in continental masses. Tanzanite crystallized at around 600 to 585 million years ago (Fig. 8). Thus, it took more than 1 billion years of geological history to form this gemstone, from the initiation of the processes that increasingly concentrated Al, Si, K and Ca, through re-melting of already crystallized rocks and the formation of rocks with an increasingly crustal nature.
To put this time frame into context let’s look at a few well known events that took place in Earth’s history (Fig. 8).
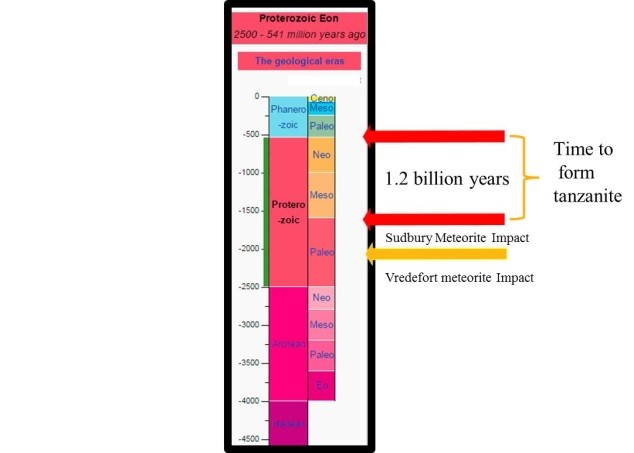
The formation of the Earth’s crust commenced round about 3.2 billion years ago. One of the major events that followed was the Vredefort meteorite impact in what is now South Africa at about 2.1 billion years followed by the Sudbury meteorite impact in Canada at about 1.85 billion years. The sediments that eventually formed the rocks surrounding tanzanite started accumulating at about 1.8 to 1.6 billion years ago and then went through several cycles of metamorphism, melting and crystallization. Atmospheric oxygen levels were slightly more than 3.1% compared to todays 21%. Life on earth was dominated by stromatolites, carbon films, eukaryotic algae and possibly red and green algae. The oldest relatively non-controversial, well-studied animal fossils appear only around 635 million years ago to 542 million years ago.
One might conclude that the gemstone tanzanite began its’ creation with the earliest life forms on earth and was finally formed simultaneously with the earliest animals.
To put this in perspective, the tanzanite that you own was already completely crystallized 148 to 135 million years before the first (at around 452 million years ago) of the Big 5 Mass extinctions (Fig. 9) on Earth and eventually the rise of Neanderthals, Denisovians and Homo Sapiens in the last million years. The descendants of the latter happen to stumble onto the gemstone 53 years ago and immediately started cutting, heating and polishing the stone. Mother nature surely sees the humour in this!
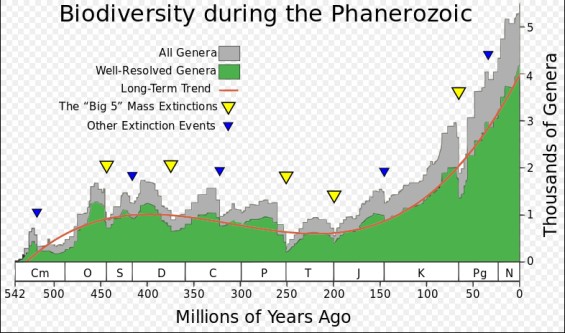
The Kilimanjaro volcanic event took place only 2.5 million years ago, thus approximately 582 million years after the crystallization of tanzanite and had NO relationship with the formation of tanzanite.
A RESTLESS Earth.
As important as time is, no tanzanite would have formed without the constant evolution of earth. When Earth formed at approximately 4,6 billion years ago it was a fairly homogeneous mass of molten rock. It took approximately 1.64 billion years for a distinct crust to develop, clearly separated from the mantle. Crustal formation was critical in the evolution of not only rocks on earth, but also evolution of animal and plant life. The main reason for this is that the process of crustal formation very effectively separated elements from those concentrating in the mantle from those in the crust via the different rock types that evolved. This process was also essential to form tanzanite.
The early crustal masses congregated in Supercontinents, an example of which is the Supercontinent Columbia (Fig. 10) which dominated during the period from 2.1 billion years to 1.5 billion years ago. These Supercontinents changed over time into different continental masses through the process of subduction whereby already formed rocks are buried and finally re-melted. Thus, by the time that tanzanite finally crystallized 600 to 585 million years ago, crustal material already went through several of these cycles.
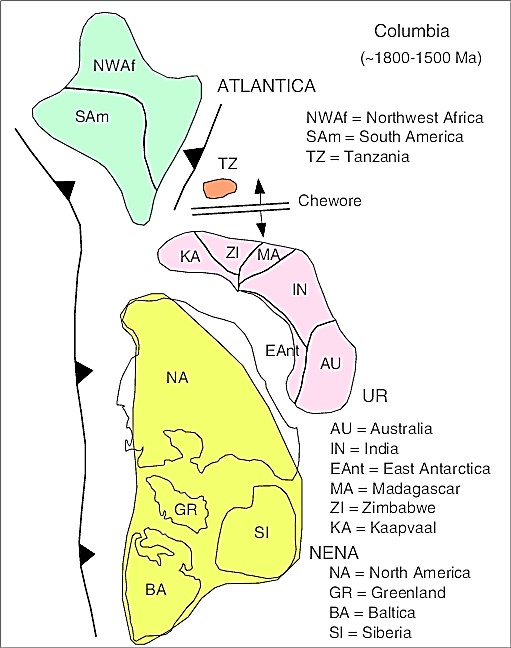
Both time and the constant evolution taking place on earth were thus absolutely essential in finally crystallizing tanzanite.
We will now look at nine basic steps that had to take place in exactly the right sequence to form tanzanite.
5. THE NINE MOST CRITICAL STEPS TO FORM TANZANITE.
Step 1. Getting Al and V together.
As illustrated above, tanzanite is the blue variety of zoisite (Fig. 11) and the key factor in obtaining the blue colour is the presence of V, an element not generally concentrated in association with an excess of Al.
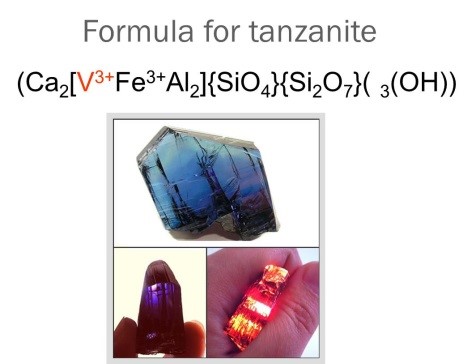
At around 850 million years ago the area which would eventually become host to the Merelani tanzanite deposit was situated in a shallow sea environment in a back arc basin (Fig. 12). The modern equivalent would be the Japanese islands and the continental mainland to the Northwest.
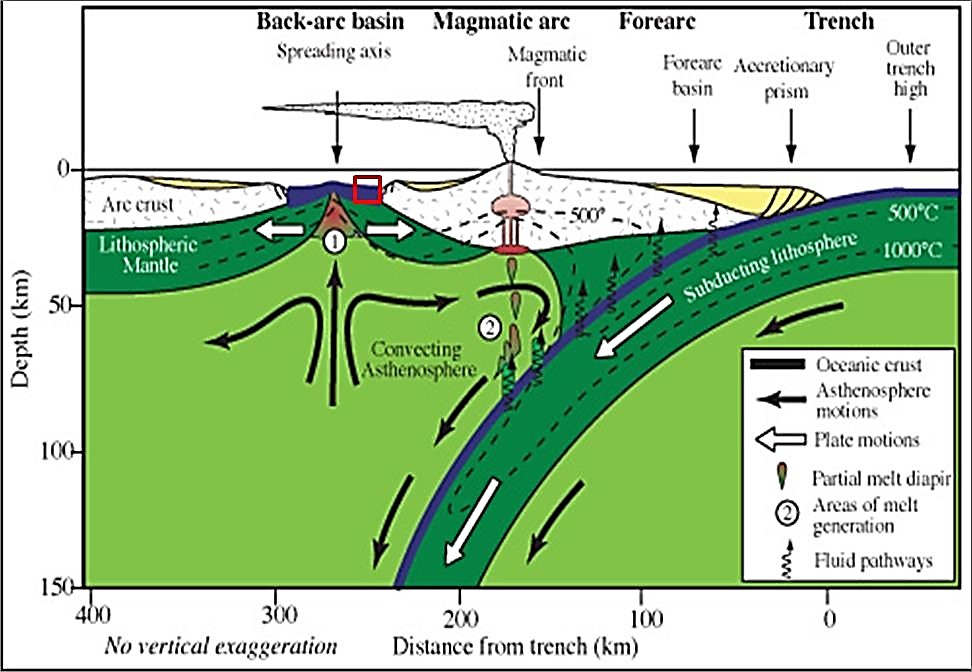
This as an ideal situation whereby Al and Si would be contributed to the back-arc sedimentary basin from the erosion of existing crustal material accumulated by sedimentation, metamorphism, re-melting and crystallization over a period of 750 million years. In addition V, Cr, Fe and Ti would be contributed to the basin waters from volcanic vents on the seafloor and weathering of existing mafic rocks.
Also important would be that carbon-rich shales (Black shales) and mud-stones would be formed as well as clay – rich sediments. The organic matter played an important role as V would be adsorbed on the organic particles. In a similar way V may be taken up in the crystal structure of Al-rich clays. This environment thus created the possibility of getting Al and V together in close proximity in a sedimentary pile. In addition Ca (also a large percentage of tanzanite’s composition) and Mg were also concentrated in close proximity to Al and V in this package of sediments as carbonate – rich rocks. This may have been achieved due to the formation of evaporate deposits. Evidence from tsavorite deposits in the Lelatema antiform suggests that sabhka deposits played a role in further concentrating Al, V, Cr, Ca, Mg and Ba (Feneyrol et. al. 2012, 2017) in the sedimentary package.
Step 1 was already extraordinary in the sense that nature provided mechanism to concentrate elements that do not normally tend to coexist in a relatively confined package of sediments. This was done by having carbon rich muds (V, Cr) as well as carbonates (Mg, Ca) and clay-rich sediments (Al) together with evaporites (Ba, Li). In addition to this seafloor vents contributed additional V and Cr.
Step 2. Further concentrating V, Ti as well as Ca and Mg and trapping the elements in rocks.
As geological time went by, the sedimentary pile got buried deeper and deeper as new sediments accumulated on top. Due to the added pressure and temperature (the geothermal gradient on continental crust is approximately 20°C per kilometer of burial) new rocks started to form during this process called diagenesis. At around 200°C, V-rich clay minerals were formed in the Al-rich sediments while at the same time a further concentration of V took place into organic rich sediments that now started to become black shales. Eventually, under increasing pressure and temperature these rocks would become hard rocks such as garnet-sillimanite gneisses, kyanite graphite gneisses and schists and dolomitic marbles.
Step 2 caused a further enrichment of the necessary elements to form tanzanite in the partly solidified sedimentary sequence by trapping the elements in suitable relatively low temperature minerals.
Step 3. Elevated temperatures and pressures.
From experimental work we know that Zoisite is not stable in Si-Al-Ca-H2O systems below at least 350°C at low pressures and at even higher temperatures at higher pressures. If nature thus intended to create tanzanite, mechanisms were needed to increase the T and P. This was achieved conveniently during the period 840 Ma to 550 Ma by the events that accompanied the so called East Africa Orogeny of which the events in the Mozambique belt are best studied. During this orogenetic (mountain building episode) a subduction event that involved subduction of Madagascar and Eastern Africa took place (Fig. 13). For a detailed discussion of the deformation of the East African Orogenic event read Fritz et.al (2013).
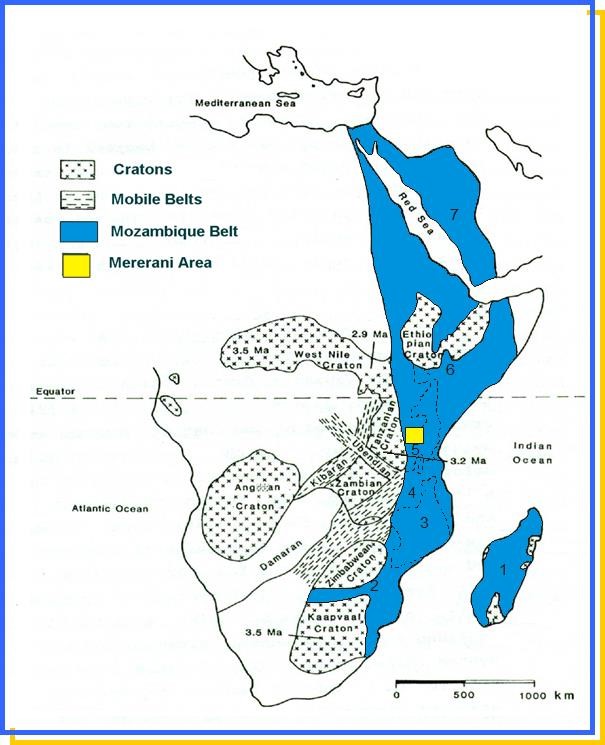
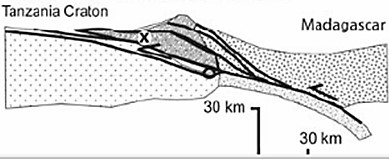
Our sedimentary pile now got subducted to depths of around 30 km deep, equivalent to immense pressures of around 10 to 12 Kb and temperatures of about 850°C (Fig. 14). The peak of this event was at around 640 Ma ago.
From a depth of around 15 km in the crust the pressure and temperature is enough for rocks to start behaving by ductile and not brittle deformation. This meant that rocks started behaving as a paste and could be deformed in incredible ways without breaking (Fig. 15).
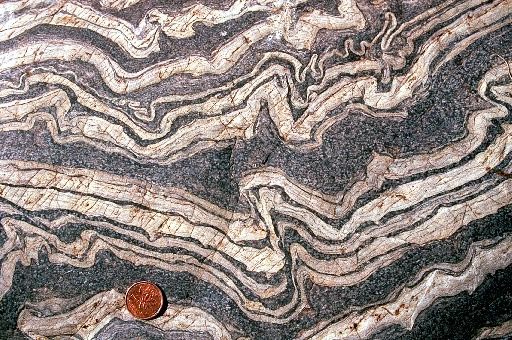
All the time new high pressure minerals such as kyanite (An Al-rich mineral) crystallized. Following the peak of the increase in P and T, was a period of pressure relief during which the pressure dropped from 10 kb to 8 kb but the temperature stayed above 800°C. At the end of this period conditions were ideal for Ca-rich garnet (grossular) to crystallize. This in turn was followed by a long time period of cooling during which the temperature was reduced rather fast (in geological time scale) but under pressure conditions that slowly decreased. As we will see this was ideal for tanzanite to form.
To achieve the temperatures and pressures that were necessary to solidify the rocks as well as to form the precursor minerals to tanzanite, we needed a subduction zone as Step 3. The subduction took place in a very specific way not to increase the pressure or temperature too fast. At exactly the appropriate conditions the rocks started to cool down again whilst maintaining a relatively high pressure for a sustained period of time. This allowed minerals to form in the correct sequence.
Step 4. Intense deformation.
As shown above, the rocks in the vicinity where tanzanite eventually formed were highly ductile during the peak of the metamorphic event. This led to intense deformation accompanying the peak metamorphic event (M1) which we may conveniently call deformation 1 (D1). The main visible feature of D1 was a folding event (F1) on an immensely large scale. To see the scale of the fold we actually need to look at a satellite image (Fig. 16).
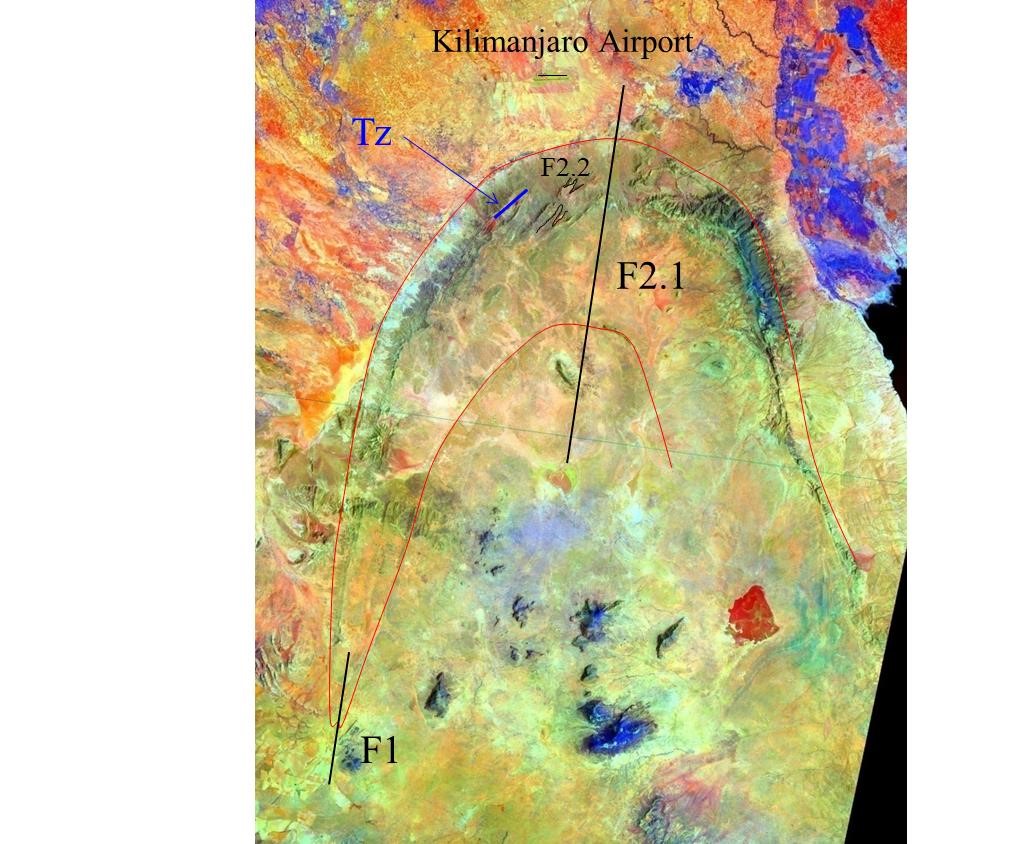
The red curve outlines the curvature of the F1 folding event where ductile deformation at around 30 km depth bent the rocks as if a flat piece of paper was folded in half. This is the lowermost left (South Western) fold on the diagram.
Next followed (under slightly lower PT conditions but still ductile deformation), the second deformation event (D2) that was accompanied by several folding events (in general F1, F2 and F3). This was the most important event controlling the crystallization of tanzanite in a spatial sense. Accompanying the D2 event we see three sub-folding events of critical importance namely:
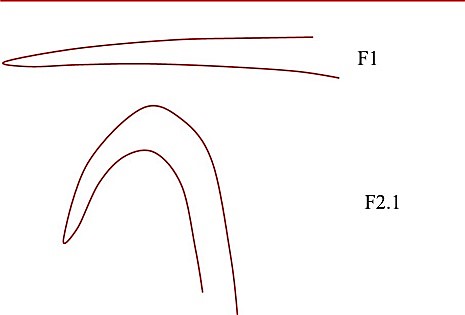
- F2.1. This event refolded the layers already folded during the F1 event. We see the expression of this as a large fold structure called the Lelatema antiform on the second fold (northern central area) on the satellite image (Fig. 16) and diagrammatically in Fig. 17).
- F2.2. Simultaneously with the F2.2 folds, secondary folds on a 150 m to 250 m scale developed on the flanks of the F2.1 folds (Fig. 18a,b).
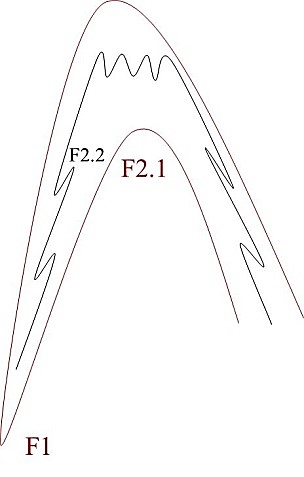
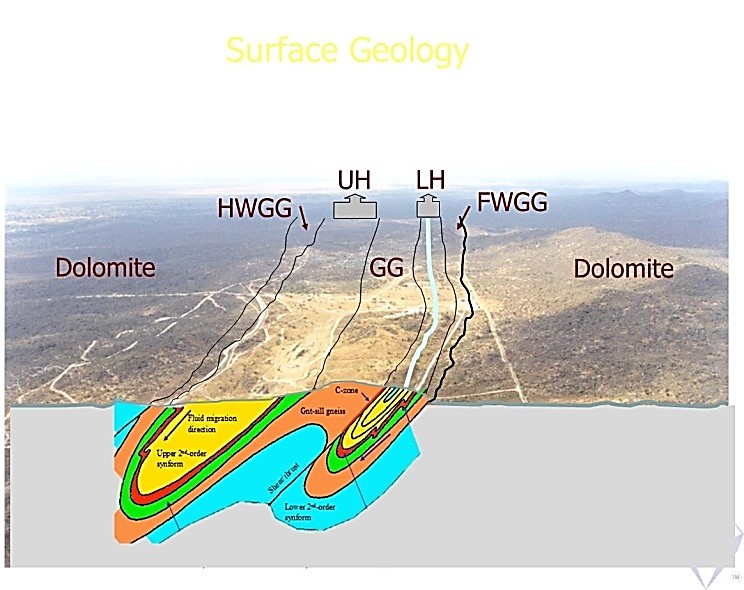
As can be seen on the above diagram these folds (F2.2) are what control the repetition of the rocks that are mined on the scale of the area in which the tanzanite mines occur.
- F2.3. On an individual mine shaft scale this is the most critical folding event of all. On the flanks of the F2.2 folds at a repetition distance of 70 m to 110 m secondary drag folds developed (Fig. 19). The P-T conditions were now sufficiently low for some rocks in the sequence to develop a brittle deformation character whilst other were still deforming ductile. A Quartz-Diopside layer occurring within the mineralized zone, as we will see later (Step 5), behaved competent at this stage. As the F2.3 fold developed on the flanks of the F2.2 folds, this competent layer broke and was piled into fold stacks at a repetition distance of about 70 m – 110 m. The rocks around the broken layer still behaved ductile and due to the stress field along the layer being folded these broken sections were separated from each other and piled on top of each other due to the folding. The F2.3 folds repeat the layers up to seven times.
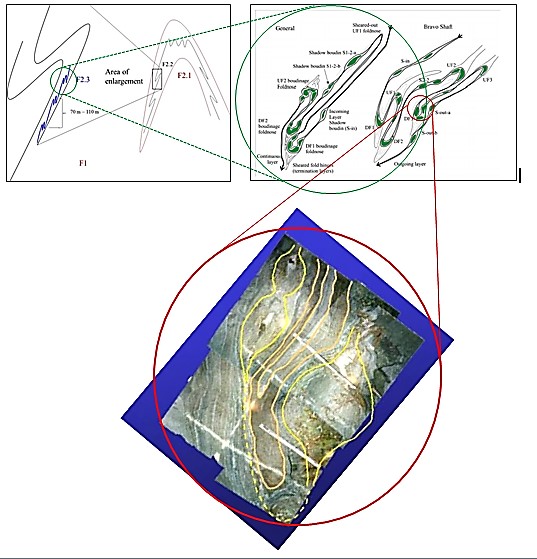
The last folding event (F3) deformed the layers containing tanzanite in a strike direction. These folds repeat every 500 m to 700 m (Fig. 20). In the diagram below, it is evident that the zones containing tanzanite (outlined in blue) are completely controlled by the deformation events and are localized to specific zones of mineralization.
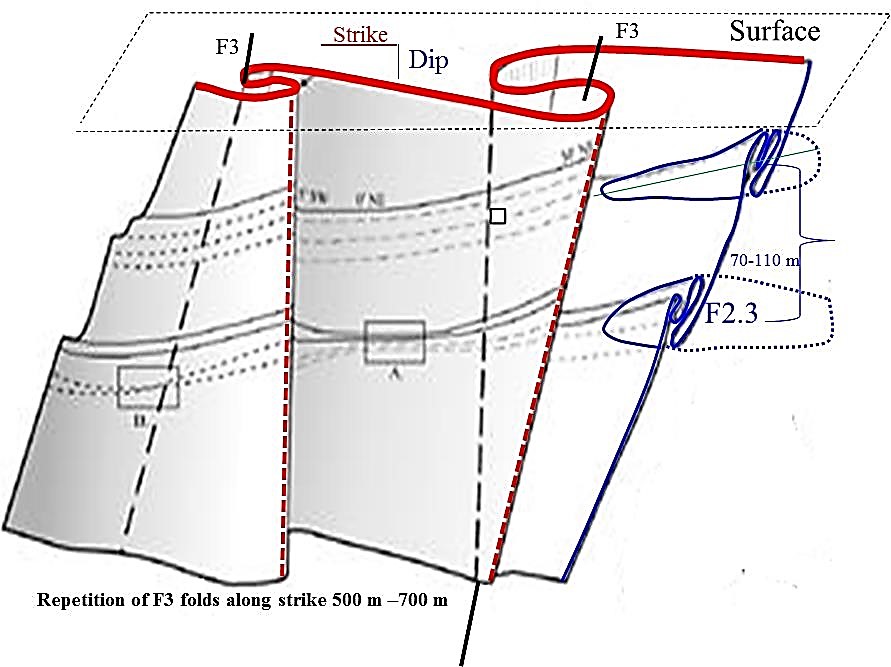
As the rocks cooled down and pressure was released on them, the ductile deformation gave way to brittle deformation. Instead of giving way by folding, zones along which rocks sheared now became possible, providing ways along which fluids could effectively move. One such a zone controlling tanzanite mineralization is a shear zone present in the JW zone that was also principally responsible for the duplication of the zone.
Following a sequence of rock-folding events from a mega- (tens of kilometers) to a meter and even centimeter scale, the trap sites for tanzanite were formed during Step 4. What makes this even more remarkable is that the formation of the trap sites and the position of the chemically suitable rocks were coinciding in space.
Step 5. Skarnification and creating local trap sites.
During or just prior to the F2.3 folding event, the competent calc-silicate (Quartz – Diopside) layer responsible for the stacking of F2.3 folds as described above also created the 5th principle mechanism for tanzanite formation. When a sequence of rocks consisting of competent and less competent rocks are put under pressure which is almost the same from two directions but higher from the third direction, structures called chocolate tablet boudins (Boudin in French = Sausage) develop (Fig. 21).
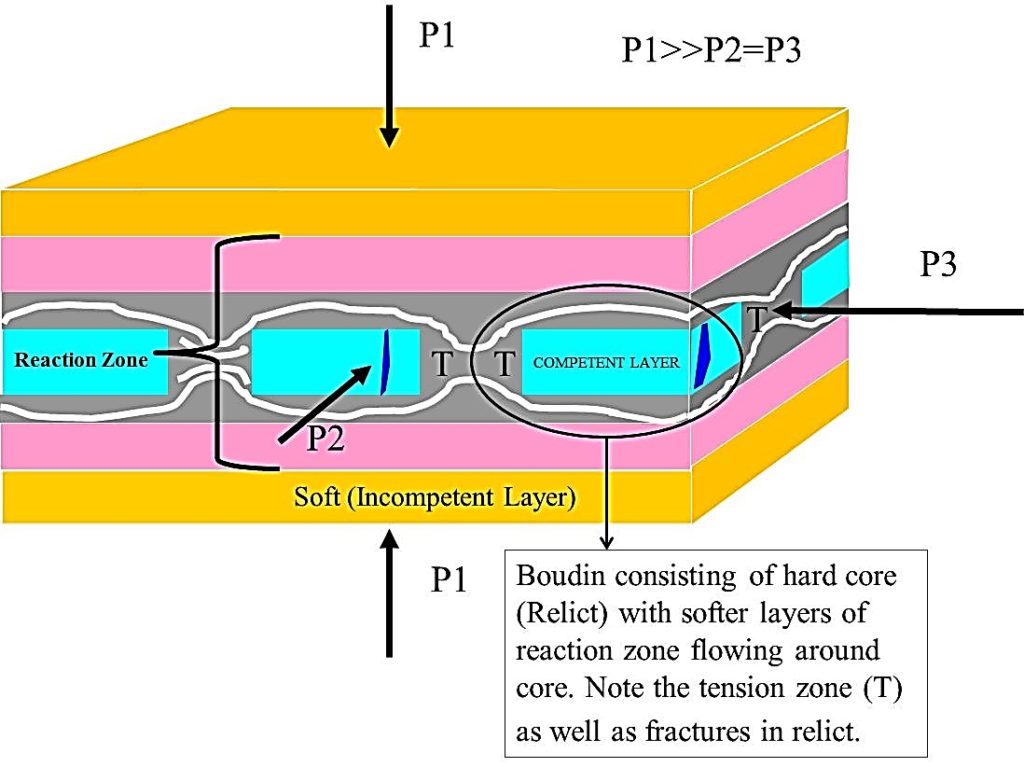
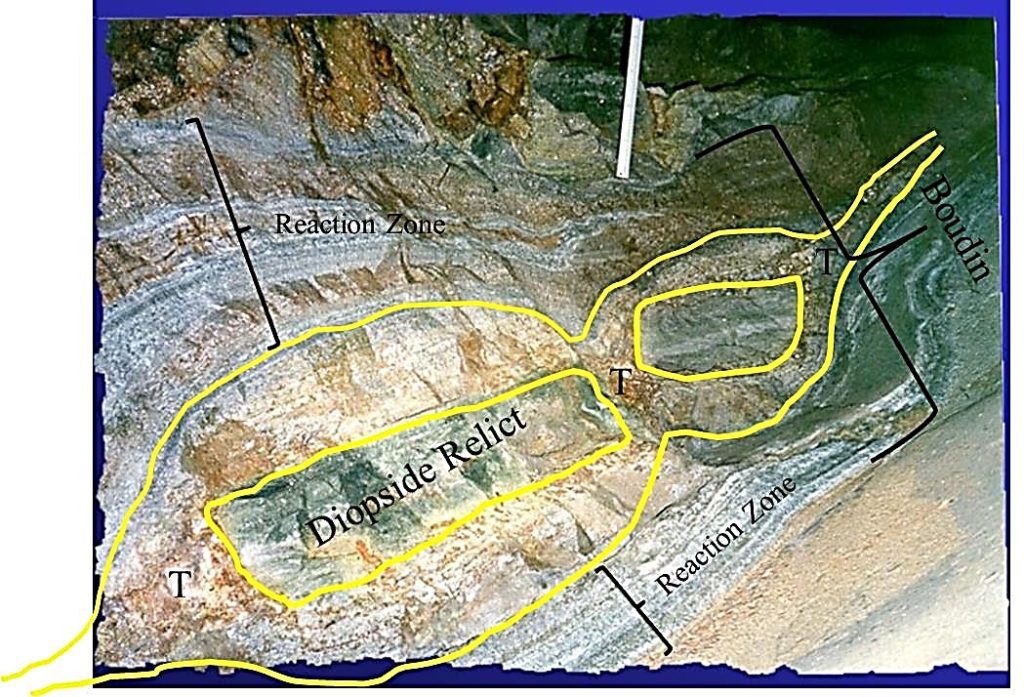
The hard (Competent Layer in Blue)) reacts by breaking under these pressure conditions forming hard cores (relicts) whilst the softer layers tend to flow around the hard relicts (Blue). The overall result of this is that tension zones (low pressure zones) are created at the edges of boudins (T) and within fractures in boudin cores (Dark Blue). The importance of this is that fluids containing elements to form tanzanite (such as V) will migrate to these tension zones. It is thus evident that these are the places that tanzanite will preferentially be found.
Chemistry and physics worked together yet again during Step 5. Chemically the Quartz – Diopside (Relict) layer formed in the sedimentary package. This layer was much harder (competent) than the surrounding rocks enabling the physical formation of boudins and tanzanite trap sites. Furthermore, the very specific condition of having the pressure almost the same from two directions, but different in a third direction was fulfilled.
Step 6. Element moving and trapping chemical reactions.
The 6th critical eventnecessary to form tanzanite was metamorphic and metasomatic reactions between the kyanite gneisses and the calc silicate units (Fig. 22).
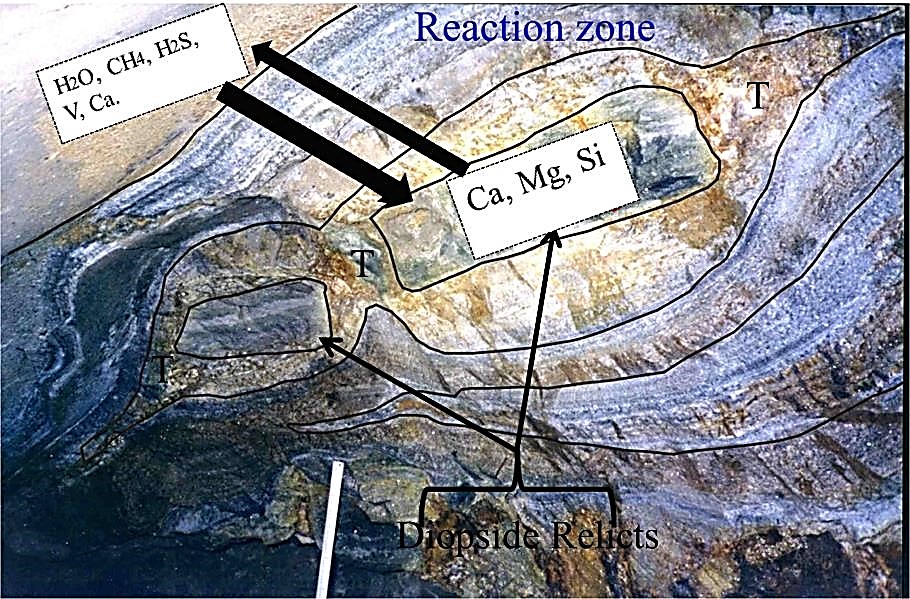
In general terms Ca and Mg migrated to the reaction zone leading to the crystallization of zoisite, grossular garnet, titanite an increased Ca content of the plagioclase and increased graphite content. A general dehydration of the surrounding gneisses and hydration of the reaction zone and boudin took place. The fluids necessary to do this was obtained by dehydration of the surrounding gneisses. This step may be considered a general preparation of the mineralization zone for the first tanzanite crystallization event.
Reactions involving fluids now became an important feature. During Step 6 the elements necessary to form tanzanite were concentrated in an around the positions where tanzanite was to be formed. Small crystals of tsavorite and other Ca-Ti rich minerals formed in the reaction zones around the bound layers.
Step 7. Cooling down and changing minerals by more reactions.
The 7th requirementto form tanzanite was aretrograde metamorphic path along which V-rich green grossular garnet (tsavorite) crystallised in tension zones within and in proximity to the boudins. During the onset of the dehydration phase (commencing during the initial phases of the retrograde phase) as described above, grossular garnet started to crystallize in the reaction zone around boudins. Further along the retrograde P-T path, during the early stage of the F2.3 folding event, grossular garnet also crystallized in the tension zones in and around boudins.
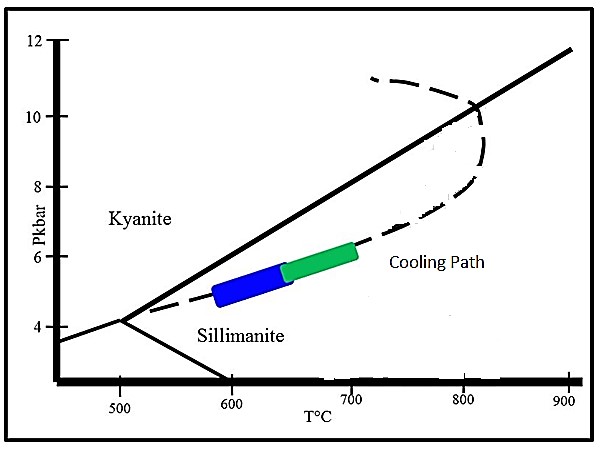
Tanzanite formation occurred during the retrograde stages at ca. 585 ± 28 Ma with P-T conditions estimated at ca. 5 to 6 kbar and 650 ± 50°C (Fig. 23).
3 Tsavorite + H2O + 5 CO2 – 2 Tanzanite + 3 Quartz + 5 Calcite
This reaction is illustrated in samples where tsavorite reacts to form tanzanite associated with quartz and calcite (Fig. 24).
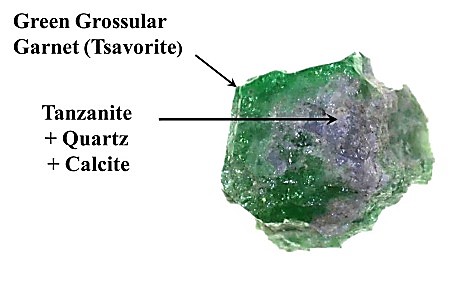
The rocks continued to cool down with a simultaneous slow release of the pressure conditions. Fluid circulation increased all the time. This enabled some mineral reactions to take place and tanzanite became a stable mineral phase. There were now enough fluids in the system to allow relatively “dry” minerals with more or less the same composition as tanzanite, namely tsavorite, to become unstable to form tanzanite (Tanzanite 1).
Step 8. Fluid Flow along brittle deformation zones.
The 8th eventto form tanzanite required for the P and T to drop further along the retrograde metamorphic path. Several events now happened in rapid succession:
Dehydration of the surrounding rocks took place at an increased rate.
The brittle component in the deformation of the rocks became increasingly important. As described above, the JW zone is essentially a shear zone (especially during F3.3) and the brittle shearing component now act as a conduit for the rapid introduction of fluids to the boudins and surrounding reaction zones. Alteration reactions now started to increase.
Early fluids may have been slightly more oxidizing in nature (containing CO2) allowing for the reaction
Tsavorite + H2O + 5 CO2 – 2 Tanzanite + 3 Quartz + 5 Calcite.
to take place.
As illustrated by electron microprobe analyses, the V content in Tsavorite and Tanzanite is roughly the same (Fig. 25).
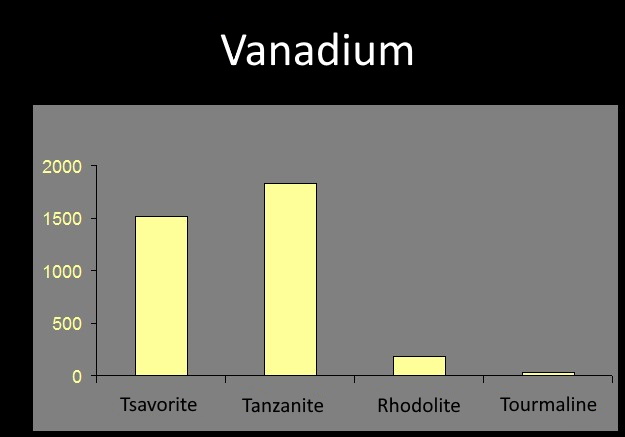
Early formed tsavorite were thus transformed to tanzanite in tension zones. This reaction is illustrated in samples where tsavorite reacts to form tanzanite (Type 1 tanzanite) associated with quartz and calcite. Note the natural blue colour of the tanzanite.
- P-T conditions further decreased and migration of fluids became increasing important along brittle shear zones and fluid migration distances increased. The fluids increasingly interacted with graphitic gneisses. As Olivier (2006) illustrated the carbon isotope signature of graphite from the Merelani deposit had an organic origin and was possibly Archaen in age (hence the long history to eventually form tanzanite). Oxygen isotope signatures of quartz and tanzanite indicate that the majority of fluids within the graphite gneisses and calc-silicates originated from the dehydration of the pelites. Giuliani et. al. (2008) and Harris et. al. (2014) pointed out that the Delta13 C value of ~-24°/°° is consistent with the source of the carbon being from a black shale. The high V values in the kyanite – graphite gneisses are consistent with a black shale protolith. In combination the C and O isotope date of Feneyrol et. al. 2017 and Harris et al. 2014 clearly support an original black shale component as crucial in eventual formation of tanzanite. These fluids (as illustrated by Olivier 2006) now consisted of H2O, CH4, H2S and N2 and were rich in V and Ca. Harris et al. (2014) pointed out that differences in δD values between tanzanite and tremolite for instance may reflect differences in water fractionation factors and a possible larger proportion of meteoric water in the case of tanzanite. V was most likely transported as V4+ to be reduced to V3+ (the chromophore providing the blue colour) within the alteration zones and specifically boudins. The fact that blue tanzanite is found in close proximity to colourles or brown tanzanite is most likely ascribed to the fact that in some instances V3+ entered the crystal structure instead of V4+ depending on the oxidation state of the fluid.
The P-T conditions for the Type 2 tanzanite formation are estimated to be ca. 6 to 5 kbar and 650 ± 50 °C.
Earlier age determinations by Fission Track dating provided an age of 585±28 Ma. Feneyrol et. al. (2017) proposed an age of 600 Ma for the tanzanites and tsavorites at Merelani based on Sm-Nd isotopic data. Although the fission track dates and the Sm-Nd dates has a large overlap, carefull selection of Type 1 and Type 2 tanzanite for dating may reflect the two different ages.
Due to the increase in available pathways for fluids to move to tension zones the necessary elements reached high enough concentrations to form new tanzanite (Tanzanite 2). The quality of tanzanite (colour, clarity etc.) was determined by the conditions in the immediate vicinity of where the tanzanite formed. More or less H2O, CO2, H2S, CH4, V3+, V4+, Ti3+ etc. were present in specific tension zones which in turn determined the oxidation state and other parameters. Each individual tanzanite will differ slightly from the next. Thus, each stone is truly unique (Tanzanite X).
Step 9. Getting to surface.
The 9th prerequisite to form tanzanite was a long period during which crustal processes and weathering brought tanzanite close to surface. Deformation processes needed to be moderate to protect tanzanite against the long – lived brittle deformation processes associated with the East African Orogeny and subsequent geological events such as rift formation. Based on current knowledge, events along the East African Rif valley (volcanism etc.) did not have any influence affecting or altering the tanzanite deposits. A detailed fluid inclusion or isotope study stage of late stage minerals might show some minor effects.
The tanzanite crystals now had to be protected where they formed deep in the crust for a period of 585 to 600 million years against the restless crustal processes taking place during normal evolutionary events on earth. Fortunately, despite the formation of the East African rift valley to their west, a few young volcanic centers some tens of kilometers away and some other minor geological events, the crystals remained intact.
Acknowledgements.
AFGEM Ltd., TanzaniteOne Ltd., Richland Resources Ltd., Tanzanite Africa Ltd. and numerous small and medium scale mine owners in Blocks A, B and D is thanked for the opportunity presented to me over a period of 18 years to visit their shafts and tanzanite workings.
Selected References.
• Feneyrol, J., Ohnenstetter, D., Giuliani, G., Fallick, A.E., Rollion-Bard, C.,Robert, J., Malisa, E. (2012). Evidence of evaporites in the genesis of the vanadian grossular ‘tsavorite’ deposit in Namalulu, Tanzania. The Canadian Mineralogist 50(3):745-
• Feneyrol, J.,Giuliani, G., Demaiffe, D., Ohnenstetter, D., Fallick, A.E., Dubessy, J., Martelat, J., Rakotondrazafy, A., Omito, E., Ichang’i, D., Nyamai, C.,Wamunyu, A. (2017). Age and Origin of the Tsavorite and Tanzanite Mineralizing Fluids in the Neoproterozoic Mozambique Metamorphic Belt. The Canadian Mineralogist. 55. 763-786.
• Fritz,H;, Abdelsalam, M., Ali, K.A., Bingen, B., Collins, A.S., Fowler, A.R., Ghebreab, W.,Hauzenberger, C.A., P.R.Johnson, P.R., Kusky, T.M., Macey, P., Muhongo, S., Stern, R.J. and Viola, G. (2013). Orogen styles in the East African Orogen: A review of the Neoproterozoic to Cambrian tectonic evolution. Journal of African Earth Sciences, 86, 65-106.
• Harris, C., Hlongwane W., Gule., N and Scheepers, R., (2014). Origin of tanzanite and associated gemstone mineralization at Merelani, Tanzania. South African Journal of Geology. 117. 15-30.
• Malisa E., Muhongo S. (1990) Tectonic setting of gemstone mineralization in the Proterozoic metamorphic terrane of the Mozambique Belt in Tanzania. Precambrian Research, Vol.46, pp.167-176.
• Olivier B., (2006). The Geology and Petrology of the Merelani tanzanite deposit. NE Tanzania. Ph.D. University of Stellenbosch, South Africa. 453 pp.
Wow! Reyno, one of the most interesting and enlightening articles I read in a long time! Certainly in the same class as the famous articles by Douglas Scholtz on the formation of magmatic granite in the days of old. Thanks for sharing.
Thank you very much Assie. Speaking of granites, I still need to publish a summary of my research on the Cape Granites. Time is just so short. At least a highlight for me was the discovery of the volcanic component of the Cape granites (the ignimbrites). These are also fascinating rocks but in a different way.