Introduction
Outcrops of small mafic to intermediate igneous rocks, gabbroic to granodioritic in composition, with or without an associated minor felsic component, occur scattered throughout the Western Cape province in South Africa. Typical occurrences are at Yzerfontein (Maske 1957), Mud River, Boterberg, Kuils River and Stellenbosch in the Tygerberg terrane (Jordaan 1990, 1995) and several occurrences in the vicinity of Malmesbury in the Swartland terrane (Fig.1) (Van Zijl 1950, Jordaan 1990, 1995). These outcrops, which are intrusive into the Malmesbury metasedimentary sequence, define a small number of medium-sized plutons.
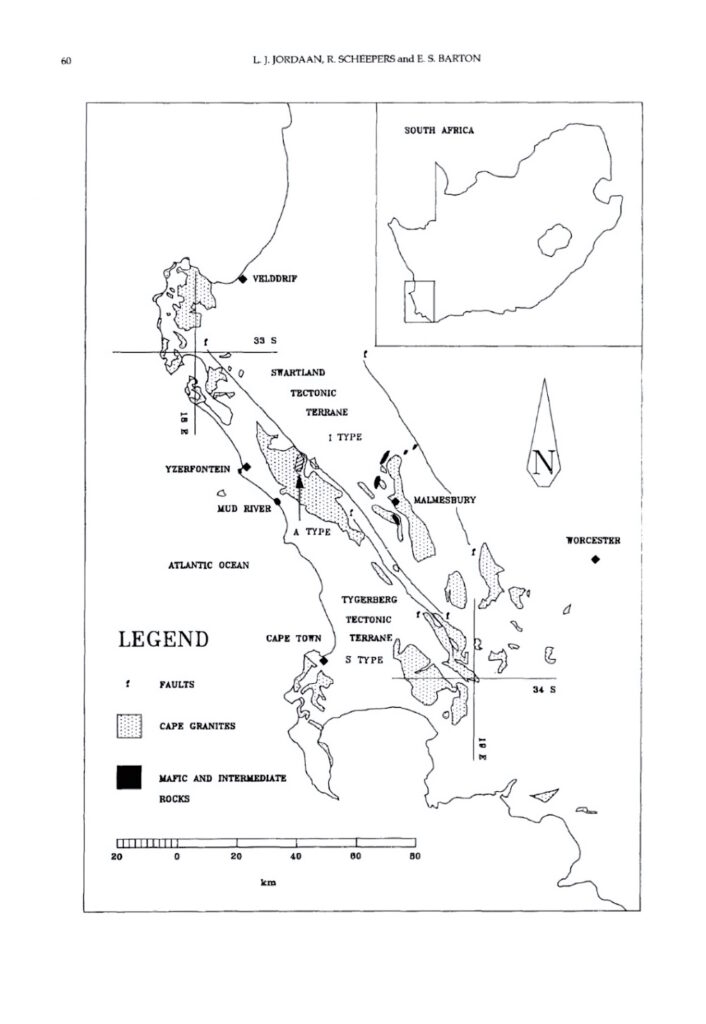
In this communication the possibility that these rocks * may convey critical information on the metallization potential of the CGS and surrounding rocks is debated.
*Please note that rock names used throughout this note are based on chemical classifications and not the IUGS rock classification system.
Current Status.
The mafic components of the Cape Granite Suite (CGS) have not been studied in detail with modern analytical techniques until the major contributions of Clemens et al. (2017) and Wilson et al. (2023) on the Yzerfontein pluton. Amongst the many significant conclusions put forward in these two publications the following are highlighted:
- An new age of ±535 Ma is proposed for the bulk of the Yzerfontein rocks, approximately 16 Ma older than the ±519 Ma age concluded by Jordaan (1990, 1995). The new age would put these rocks well within the major phase of S- and I- type granitic plutonism as well as slightly younger (±5 Ma) than the felsic volcanism related to CGS. Clemens et al. (2017) noted the complex nature of zircons in these rocks and invoked zircon mixing of zircons derived from granitic melts (from the Malmesbury metasediments) and incorporated in the mafic melts. This deduction would also assist in the explanation of a crustal signature present in the mafic rocks, although elaborate explanation of the significant amount of crustal zircon versus a small amount of crustal mixing necessary to explain the chemistry of the mafic rocks is necessary. In addition, Hf heterogeneity similarities between the S-type CGS rocks and the Hf in the Yzerfontein melts is used to substantiate the conclusion of the incorporation of Malmesbury derived crustal material.
- Scheepers (1990) illustrated the highly potassic nature of SUBALKALINE metaluminous Cape granites and Jordaan (1995) labeled the Yzerfontein “suite” High – K calc – alkaline. Clemens et al. (2017), using up to date analytical techniques, convincingly illustrated that the Yzerfontein pluton is actually shoshonitic in nature. This conclusion undoubtedly has implications for the nature of these plutons in terms of their mineralization potential.
- Clemens et al. (2017) also concluded that there is no relationship between the Yzerfontein pluton and I-type granites of the CGS, in contrast to the suggestion of Scheepers (1995), the latter also hinting at a relationship between these rocks and A-type granites of the CGS. I-type granites predominantly occur in the Swartland terrane, which make their proposed relation with the Yzerfontein rocks complicated to explain in any event.
- Importantly, Clemens at al. (2017) concluded that the Yzerfontein rocks were derived from partial melting of fertile, metasomatically enriched mantle with possible contribution from a sediment blanketed oceanic slab. These mantle derived magmas provided the heat for the Malmesbury rocks to reach granulite facies metamorphism with melting in places to produce the felsic plutonic and volcanic rocks of the CGS. If the scenario proposed in d) is correct, mafic rocks at least ±17 Ma older than the Yzerfontein pluton at ± 535 Ma must be present as the oldest S-type CGS granite dated is the Hoedjiespunt granite at ± 552 Ma (Scheepers and Armstrong 2002)) or the date of the Hoedjiespunt granite must be re-visited.
- Based on fluid inclusions, δ18O, δD and εNd data, Wilson et al. (2023) concluded that the mineralized veins present in the Yzerfontein pluton (Fig. 2) presents a shallow epithermal system related to meteoric fluids with little or no contribution of magmatic fluids at temperature conditions of 170°C to 300°C and pressures of no more than 1.6 kb. Nonetheless, Au values of up to 12 g/t have been reported in some of the veins (Wilson et al 2023). Under the current model this gold must have been transported (most likely from within the pluton) under post solidus conditions by meteoric fluids with a NNW – SSE dominant direction.
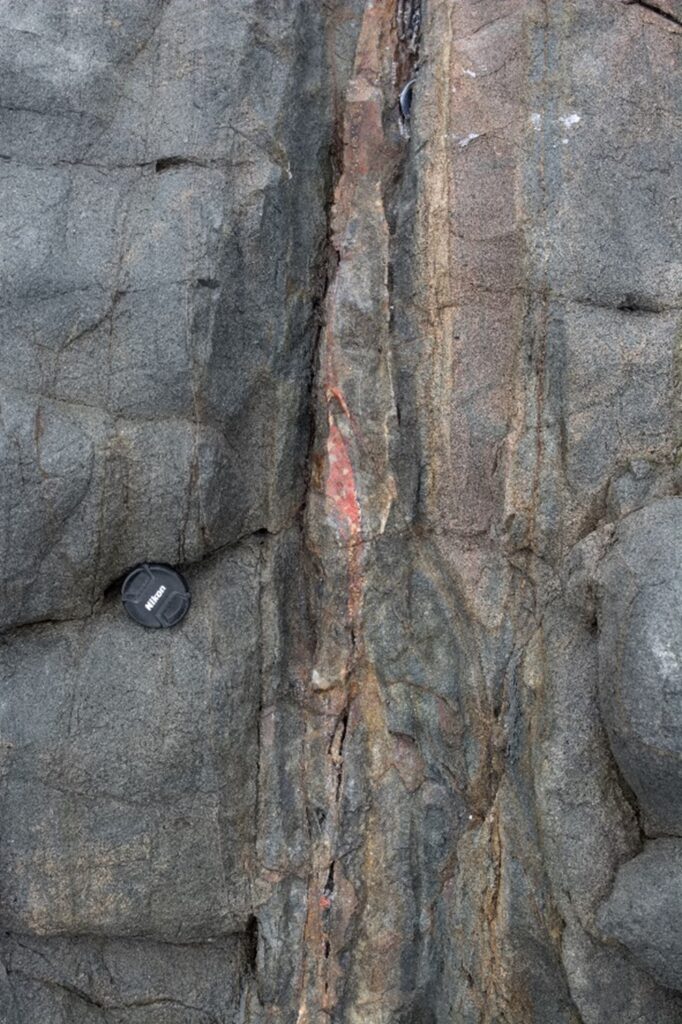
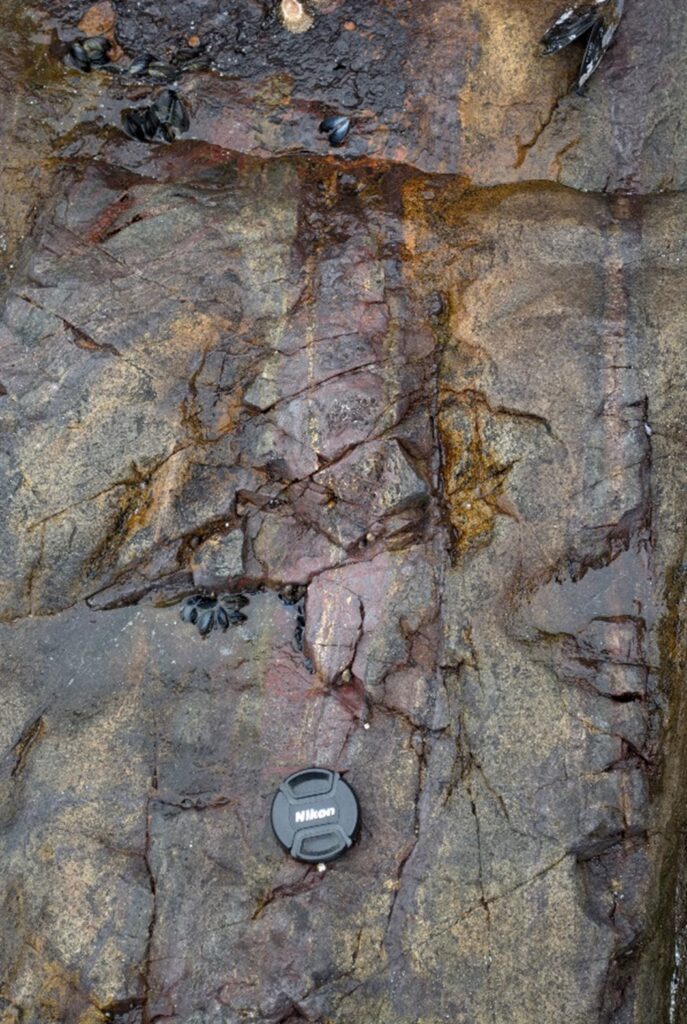
The significance of enclaves in quartz syenite dykes.
In a SiO2 against K2O plot (Fig. 3) of mafic, intermediate and felsic plutons in the western portion (Fig. 1) of the CGS some relevant observations can be made:
- Rocks of the Yzerfontein pluton (red open diamonds) based on the data of Jordaan (1995) plot well within the shoshonitic field supporting the high-quality data published by Clemens et al. (2017).
- Enclaves within the quartz syenite dykes mapped by Jordaan (1995) and studied by Slabber (1993, 1995) (Fig. 4) illustrated that rocks with chemical compositions ranging from pyroxenite to syenite are present in the quartz syenite dykes. The Ti/100 – Zr – Y diagram in Fig. 5 reveals an overlap of the enclave compositions and the surrounding Yzerfontein rocks (enclave samples red squares and surrounding rocks green and blue symbols in Fig. 5). On the SiO2 – K2O diagram the enclave compositions also plot as shoshonitic.
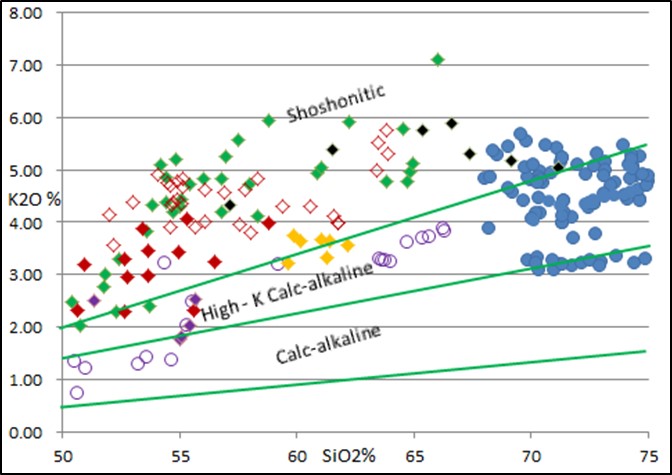
c) The Mud River pluton (red filled diamonds) is plotting well within the shoshonite field whereas the Boterberg pluton (orange filled diamonds) straddles the boundary between shoshonitic and High – K calc – alkaline.
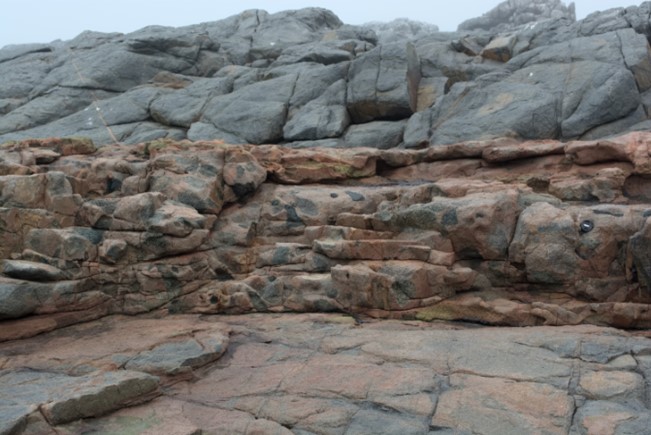
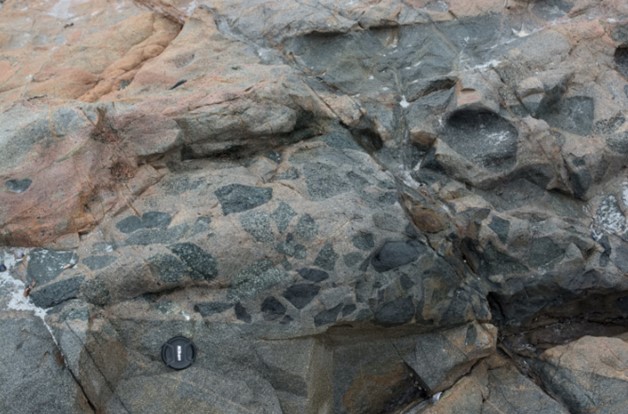
d) Rocks of the Malmesbury pluton (purple open circles on Fig. 3) plot exclusively as Calc alkaline to High – K calc – alkaline.
e) The Alexanderfontein syenites and quartz syenites (Scheepers 1982) plot as black diamonds on Fig. 3 and fall within the shoshonite field.
f) It may be concluded that mafic to intermediate rocks of the CGS sampled from the Tygerberg terrane have a shoshonitic affinity whereas those of the Swartland terrane have a High – K calc – alkaline affinity.
g) Granitic rocks sampled in the Swartland terrane with I-type characteristics cross the boundary between High – K Calc – alkaline and shoshonitic (blue filled circles Fig. 3).
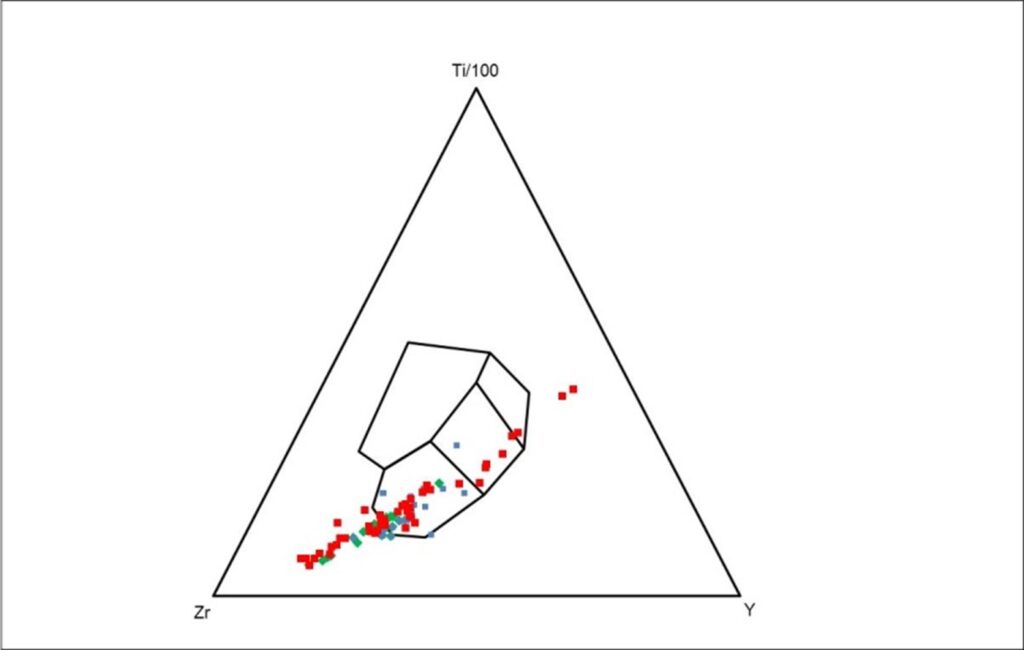
From the above deliberation it may be concluded that rocks with a wide variety in chemical composition from ultramafic to felsic underlie the Yzerfontein pluton and were transported by late quartz syenite dykes into the pluton. There is also a clear difference between the mafic and intermediate series rocks in the Tygerberg terrane and those in the Swartland terrane.
Discussion
Wilson et al. (2023) illustrated that the mineralized veins in the Yzerfontein pluton is most likely related to a low temperature epithermal event with the bulk of the fluids meteoric in origin. The structural setting of these veins also suggests, at least partially, a low -pressure regime during their formation, most likely brittle conditions during the waning post-solidus cooling phase of the pluton. The ± NW – SE dominance of the vein system coincides with the main structural trend of plutonic rocks in the Western Cape.
What may not be evident from previous publications is the extent of the veins and related shear zones. In Fig. 6 two examples (of many parallel shears) are provided of 3 to 5 m wide shear zones. In Fig. 6 a) material along the shear has been weathered out (most likely consisting of a majority of calcite, epidote and chloritized brecciated quartz monzonitic material) and in Fig. 6 b) a 3 to 5 m weather resistant shear containing mostly brecciated jasper, and chloritized quartz monzonite is shown.
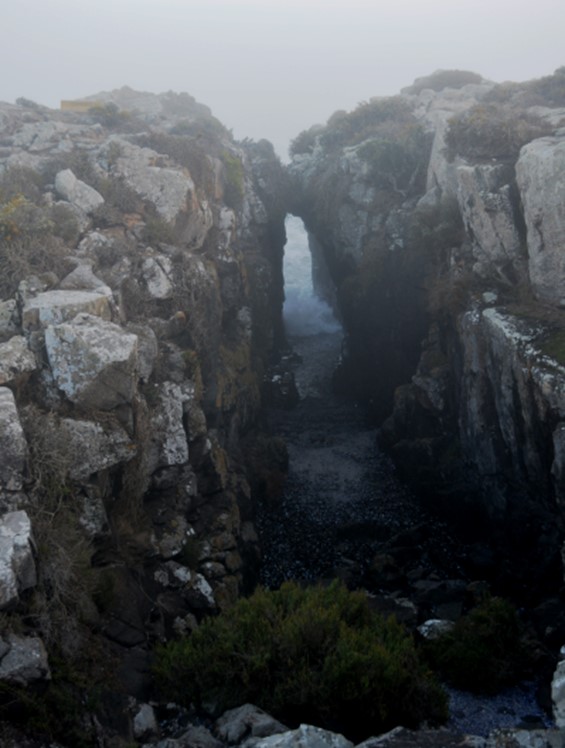
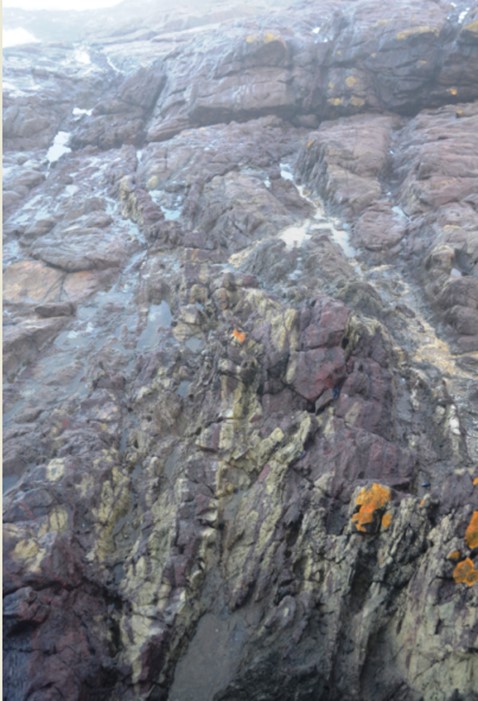
As pointed out by Wilson (2023) the direction of the hydrothermal veins seems to be parallel to major shear directions in the western portion of the CGS. The shear zones were active over a significant time period and as argued below, from ductile to brittle conditions. The shear zones are clearly showing evidence of brittle condition shearing with possible “Riedel” shearing (Fig. 7), the shears filled with jasper and tourmaline.
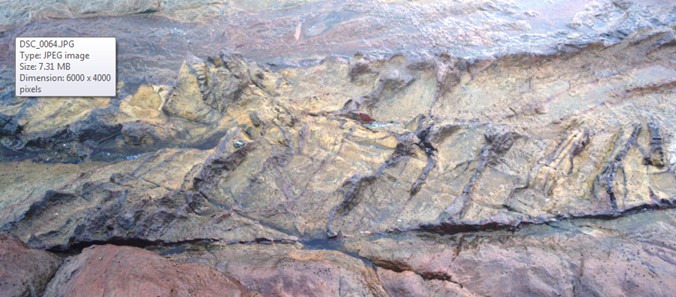
On closer inspection of some shears though (Fig. 8), evidence of ductile deformation around a fragment is evident. The structure is clearly disrupted by later events.
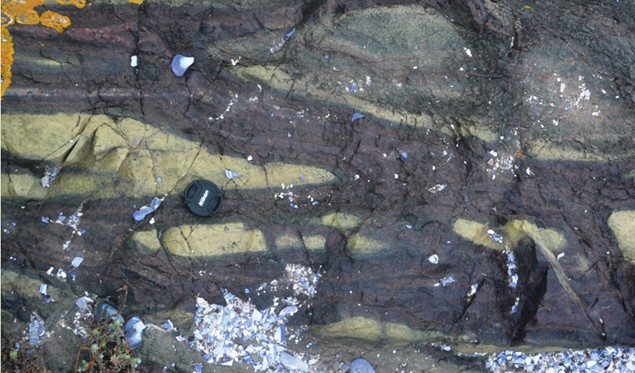
Also suggesting potential ductile deformation of layers along the shears is provided by with what appears to be evidence of boudinage (Fig. 9). In the example in Fig. 9 a), layers flow around a central core to meet in the “boudin neck” on the left. In the example in Fig. 9 b) the central boudin core is surrounded by material (darker) that also fills the fractured (stretched) core of the boudin.
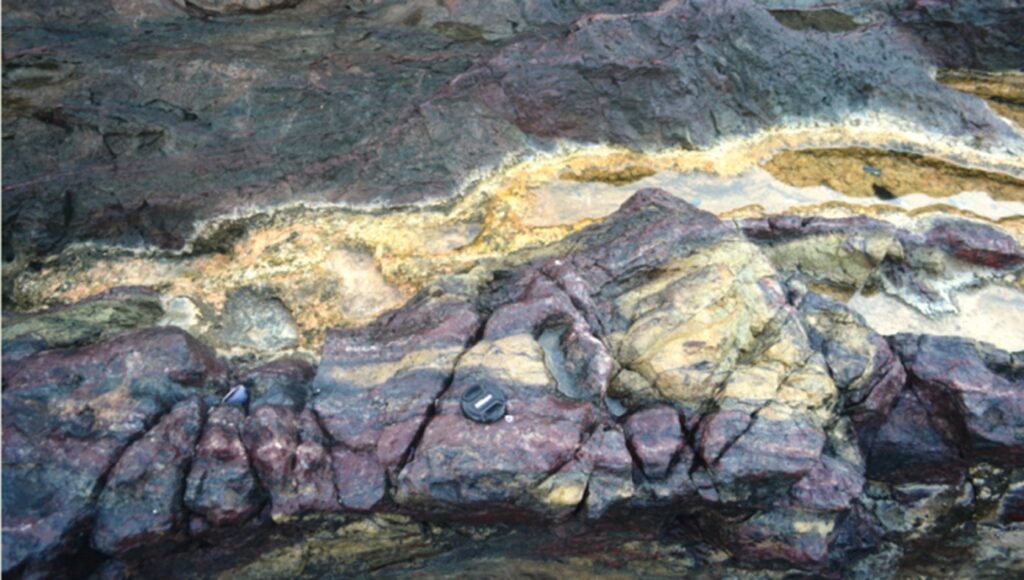
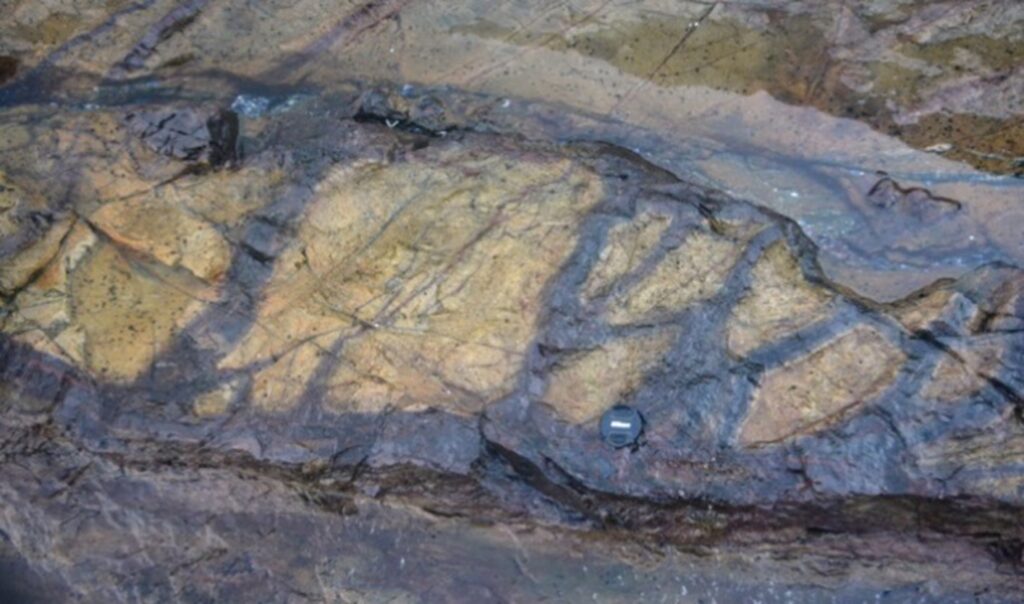
Perhaps the most significant evidence of these shears being active over an extended time period is provided in Fig. 10. In this example we see:
- Quartz syenite emplaced along a shear zone (the shear thus acting as a channel for magma emplacement).
- Evidence of rounding of rock fragments as well as angular fragments in close proximity with each other suggesting rapid (even catastrophic) emplacement (notice filled fractures in rounded fragment) of magma under high fluid pressure conditions into a lower pressure regime.
- Most significantly, previously sheared and altered fragments, now rounded, being carried by quartz syenite magma (yellow rounded pieces for instance next to camera lens cap).
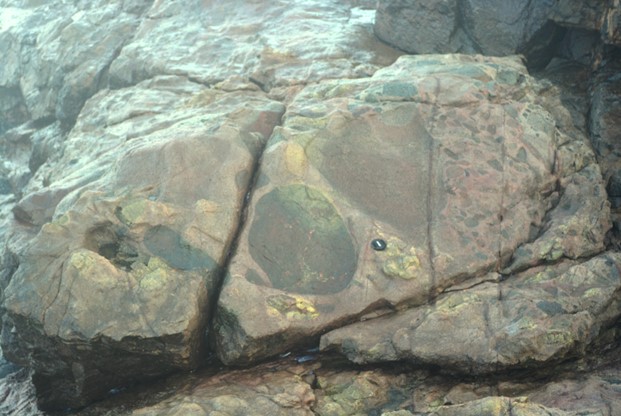
All of the above seem to indicate towards the possibility that these shears were active over an extended period of time range from ductile to brittle conditions. Although debatable, there is evidence of fluid overpressure along some shears resulting in fragmentation filled by quartz syenite and in some cases tourmaline.
It may thus be argued that these shears are not only affecting the pluton during post solidus conditions but indeed played a role during the emplacement of the magma.
A sequence of events may be:
- Emplacement of shoshonitic magma into the lower crust.
- Movement of magma along pathways created by NW-SE shearing.
- Forming of various rock types as a result of fractional crystallization and contamination with lower Malmesbury Group.
- Emplacement of the Yzerfontein magma into upper crust along shears and crystallization of the upper portions of the magma.
- Brittle shearing and epithermal alteration along shear zones.
- Fluid overpressure along portions of shear zones, disrupting previously altered rock and emplacement of fractionated magma such as quartz syenites.
- Continued shearing and epithermal alteration along shear zones.
As discussed above, the mafic to intermediate to felsic (quartz syenite) rock series of at least the Tygerberg terrane originated from fertile metasomatized mantle material with or without oceanic crust in the melt and with some crustal contamination (Malmesbury Group). In addition, it seems to be evident that although only small volumes of these rocks currently outcrop, they are underlain by large volumes of mafic to intermediate plutons. These rocks are shoshonitic in nature. At least two of the studied plutons are enriched in Cu and Au and are hydrothermally altered as proven under low pressure conditions and mainly by meteoric fluids. Evidence of fluid overpressure and related hydrothermally induced brecciation are evident in the Yzerfontein diorite (Fig. 10). Although more work needs to be conducted on this aspect there is some evidence to indicate that local overpressure along the NW-SE shear zones produced brecciation with accompanying quartz emplacement and in places tourmalinization.
Mineralization associated with High – K calc – alkaline and shoshonitic magmatism.
Ore deposits are increasingly considered as expressions of depositional points along specific mantle to upper crust pathways of magmas and hydrothermal fluids concentrating the metallogenic budget made available through variably enriched (“fertile”) mantle.
Rocks belonging to the shoshonitic series are known to have Cu – Au porphyry related deposits, an example being the Cadia Cu – Au deposits in the Eastern Lachlan fold belt in Australia (Holliday et al. 2002, Chhun 2004). All of these porphyry deposits show a close spatial association with shoshonitic monzodiorite to quartz monzonite dykes and stocks of the Cadia Intrusive Complex (CIC). In contrast, sub-economic Cu–Au mineralization at Cargo and Copper Hill in the fold belt is associated with calc-alkaline quartz diorite and dacitic intrusions. The clear – cut difference between S- and I-type granites in the Lachland fold belt as well as the granites in the Western Cape, both divided by a well-defined shear zone, is of note. Although there are differences between the LFB and the CGS, there are also many similarities.
Many other examples linking Cu-Au deposits with shoshonitic magmatism is known. An interesting example is the metal deposits of Sulawesi in Indonesia (Van Leeuwen and Pieters (2011). In this case:
- Northern Sulawesi consists of calc – alkaline series rocks contain amongst other intrusion related base metal Au and skarn deposits. Interestingly, some of the Cu-Au (±Mo) mineralization is found in tourmalinized breccias in diorite (Dunu deposit).
- Western Sulawesi consists of high-K calc-alkaline to ultrapotassic igneous rocks with porphyry Mo, porphyry Cu ± Au and are intrusion related. The rocks comprising the West Sulawesi Suite (WSS) consists of granite, monzonite, quartz monzonite and granodiorite with dioritic enclaves showing metaluminous I-type and mostly ilmenite – series granitic rocks which represents continental margin, high – K calc-alkaline to shoshonitic series in an extensional, post-subduction tectonic setting. Most interestingly the High – K (shoshonitic)‐ series magmatism produced large volumes of granitoids and subordinate amounts of tuffs that were exclusively felsic in nature and of high‐K calc‐alkaline composition (similarity to CGS?).
It needs to be pointed out that in the case of the CGS, I-type granites are related to the magnetite series and not the ilmenite (reduced) series.
Baker et al. (2016) describe the important (16.8 Moz Au) Kis¸ladag˘ high-K calc-alkaline to shoshonitic porphyry Au deposit, which formed at ~14.5 Ma in a post -collisional extensional setting within the Menderes massif of western Anatolia.
A recent (2016) Special Publication (number 19) of the Society of Economic Geologist describes numerous examples of High – K calc-alkaline and shoshonitic magmatism associated with mineralization in a young subduction setting, the Tethyan Orogenic Belt. Although outside the scope of this communication, the articles in this publication describes the intricacies associated with these deposits in fascinating detail including “the transition from collisional to post-collisional extensional and trans-tensional tectonics, the evolution of half-graben volcano-sedimentary basins and their control on the formation of high- and low-sulfidation epithermal vein systems, and deeper-seated porphyry systems”.
A last example of shoshonitic rocks in an older setting (Grenvillian) and the evolution to granitic compositions is the Eastern Chhotanagpur Gneissic Complex (Goswami and Bhattacharyya 2014).
Although the more recent (younger) examples shown above are of interest in studying unaltered (by recent tectonics) examples of shoshonitic related metallization it is the similarities between the CGS rocks and the LFB in Australia that should be pursued in terms of understanding potential mineralization related to the CGS.
Exploration
Pertinent questions that need to be addressed include:
- If the I-type granites of the CGS are not related to the shoshonitic series in the Tygerberg terrane, are they related to the high – K calc – alkaline series of the Swartland terrane? In a geographical sense they obviously are.
- Does the Riviera W-Mo (REE) enriched pluton belong to the high – K calc – alkaline series of the Swartland terrane or the shoshonitic series of the Tygerberg terrane or is the Riviera pluton in a class of its own?
- Are the volcanics in the Tygerberg terrane related to the shoshonitic series rocks as is the case in Western Sulawesi or are they simply produced from remelting of lower Malmesbury Group by intrusion of mafic magma in the lower crust with no relationship with the mafic and intermediate intrusions?
- If the granites of the CGS are produced by melting of lower Malmesbury Group rocks, what are the compositions of these rocks (Malmesbury) and are they fertile in a metallization sense. In other words, were they capable of producing melt material capable of enriching granites in economic quantities of W-Mo-REE-Au and Cu? The contribution of Slabber (1995) on the Bridgetown basalts in this regard is of interest and significance.
- If all the CGS granites were produced by melting of lower Malmesbury with little mantle contribution, what induced metallization of the felsic components of the suite such as Riviera W-Mo (REE), Mo in the Wolwefontein quartz porphyry and Cu-Mo (±Au) in the Alexanderfontein breccia pipes?
- Where do the I-type granites of the so-called minor plutons of the CGS (Robertson etc.) fit in and where are the mafic and intermediate rocks related to these plutons? Granite outcrops are found as far east as Sardinia Bay. How many unknown plutons are there? Ditto for the S-type granites of the eastern plutons.
Of critical importance to answering the above questions is:
- An understanding of the tectonics of not only the western portion of the CGS but also the eastern part at least as far as Knysna. High quality geophysical data would be essential.
- High quality modern geochemistry on all the plutons of the CGS. In a forthcoming discussion an attempt will be made to use a synthesis of available data to put the various granites as far east as Knysna in context with the rest of the CGS.
- Where is the Cu ± Au in the Yzerfontein, Mud River and Boterberg plutons from? As shown for the Yzerfontein pluton the mineralization is epithermal – did this epithermal system pick up the Cu ± Au from the shoshonitic contribution from the mantle or from the surrounding Malmesbury rocks? This is a critical question in relation to the fact that shoshonitic magmas do have an affinity for enrichment in these elements.
- The role of not only the Colenso shear zone but the plethora of parallel shear zones should be investigated. Did the mafic and intermediate plutons only act as a heat source to get epithermal systems active or are they mineralized a significant distance away from the plutons as well?
As clearly exemplified by work done on recent settings and metal deposition such as the Tethyan Orogenic belt, an understanding of the underlying structural and geochemical evolution over time for the CGS and surrounding rocks would be essential to understand potential metallization.
Are both S- and I-type granites sourced from the Malmesbury Group with the mafic and intermediate rocks simply contributing in their genesis in the form of heat supply?
Are there granites with a mixed mantle / Malmesbury origin and how do they differ from the rest?
Disclaimer.
This communication should in no sense be interpreted as a suggestion, appeal or any other form of enticement to conduct mining or exploration in the vicinity of the Yzerfontein – Mud River – Boterberg area. Its sole attempt is to contribute towards a potential model of understanding mineralization associated with High – calc – alkaline and shoshonitic mineralization associated with the CGS.
Article written by Dr. Reyno Scheepers
References.
Chhun E. (2004). Ordovician igneous rocks of the central Lachlan Fold Belt: Geochemical signatures of ore-related magmas. M.Sc. thesis. University of Sydney. School of Geosciences.80 pp.
Clemens J.D.’,Buick I.S., Frei D., Lana C., Villaros A. (2017). Post-Orogenic shoshonitic magmas of the Yzerfontein pluton, South Africa: the ‘smoking gun’ of mantle melting and crustal growth during Cape granite genesis? Contr. Miner. Petr.172:72, 24 pp.
Goswami B. and C. Bhattacharyya. (2014). Petrogenesis of shoshonitic granitoids, eastern India: Implications for the late Grenville post-collisional magmatism. Geoscience Frontiers. 5. Issue 6. P. 821 – 843.
Holliday J.R., Wilson A., Blevin P.L., Tedder I. (2002). Porphyry gold-copper mineralisation in the Cadia district, Eastern Lachlan Fold Belt, New South Wales, and its relationship to shoshonitic magmatism. Mineralium Deposits 37(1): 100 – 116.
Jébrak M. (1997). Hydrothermal breccias in vein-type ore deposits: A review of mechanisms, morphology and size distribution. Ore Geology Reviews 12. P 111-134.
Peccerillo, A. and Taylor, S.R., 1976. Geochemistry of Eocene Calc-alkaline Volcanic
Rocks from the Kastamonu Area, Northern Turkey. Contrib. Mineral Petrol 58: p.63-81.
Jordaan L.J. (1990): The Geology and geochemistry of mafic and intermediate igneous rocks associated with the Cape Granites. M.Sc. thesis. University of Stellenbosch. 216 pp.
Jordaan L.J., R. Scheepers and E. Barton (1995). Geochemistry and isotope composition of the mafic and intermediate igneous components of the Cape Granite Suite. Jnl. Afr. Earth Sci., 21(1), 59-70.
Maske S. (1957). The diorites of Yzerfontein, Darling, Cape Province. Ann. Univ. Stellenbosch, 33 Sec A 1-11, 3 – 68.
Scheepers R. (1990). Magmatic associations and radio-element geochemistry of selected Cape Granites with special reference to sub alkaline and leucograntic phases. (In Afrikaans). University of Stellenbosch. 151.pp
Scheepers R. (1995). Geology, Geochemistry and petrogenesis of late Precambrian S, I and A-type granitoids in the Saldania Mobile Belt, Southwestern Cape Province. Jnl. Afr. Earth Sci. 21(1), 35-58.
Slabber N. (1993). Quartz syentite dykes and their enclaves in the Yzerfontein diorite. Unpublished Hons. Project. University of Stellenbosch. 35 pp.
Slabber N. (1995) The Geology and geochemistry of the Bridgetown Formation of the Malmesbury Group, Western Cape Province, South Africa. M.Sc. thesis. University of Stellenbosch. 186 pp.
Van Zijl P.J. (1950). The complex dioritic stocks west of the Malmesbury – Paardeberg Granite pluton. Ann. Univ. Stell. Xxviii, 69-72.
Wilson S.; R. Bailie; C. Harris; S. Dunn; V. Silinda; S.N. Lembede (2023). The origin, nature and fluid characteristics of the hydrothermal veining crosscutting the Yzerfontein gabbro-diorite, Cape Granite Suite, South Africa. South African Journal of Geology (2023) 126 (3): 275–294.